Gems Recovered from Sedimentary Rocks
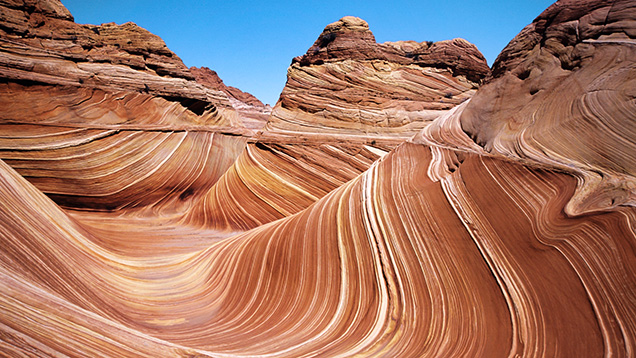
Sedimentary rocks such as shale, limestone, and sandstone are among the most common rocks exposed on the earth’s surface (figure 1). In actuality they represent only a thin covering of the crust, which mainly consists of igneous and metamorphic rocks. Despite their relatively insignificant volume, many of the major geologic events in the earth’s history are more accurately documented and age-dated by their scientific study. In addition to having economic deposits of oil, natural gas, coal, and certain heavy minerals such as gold and platinum, sedimentary rocks also host important primary and secondary occurrences of many gemstones (Dill, 2018).



The occurrences of gems in sedimentary rocks result from two different geological processes. Opal and turquoise, for example, can form in voids or empty spaces by precipitation from water or mineralized solutions, respectively (figure 2). In contrast, other gems such as diamond and sapphire were weathered out of their original igneous or metamorphic host rocks and then carried away by erosion to be later trapped in sediments. Their hardness and resistance to mechanical abrasion allowed these minerals to be transported and then deposited by moving water to create alluvial or placer gem deposits (figures 3 and 4). Both categories of gems in sediments will be discussed in this column.
SEDIMENTARY ROCK FORMATION
A three-step sequence of geologic processes results in sedimentary rocks forming on or near the earth’s surface. The first step is termed weathering—the physical, chemical, and biological decomposition of preexisting rocks of all kinds to form finer-sized particles. These particles accumulate and compact in geological basins over time to form new sedimentary rocks. Weathering involves the combined actions of air, water, chemical salts and/or solutions, freezing and thawing temperature cycles, and the effects of plants and animals on rocks.
The change in relative abundance of the various minerals from the original rock to the final sedimentary products is a measure of their comparative resistance to weathering. In our Winter 2022 column, titled “Gems Formed in Magmatic Rocks,” we discussed the concept of “Bowen’s reaction series” (figure 7 in Palke and Shigley, 2022). Based on field observations of rock relationships and experimental studies of their formation, this concept describes the sequence of formation of the common rock-forming silicate minerals at specific decreasing temperatures from a cooling magma.
In a similar way, geologists have recognized a “mineral dissolution series” of the relative stability (or “resistance to weathering”) of common minerals at the earth’s surface (figure 5). This dissolution series is also based on several other factors, including the extent of bonding of silica tetrahedra in the mineral’s crystal structures, as well as the relative resistance of their chemical elements to leaching by water. Minerals that are more resistant to weathering and dissolution are more likely to be retained in sedimentary environments and concentrated in alluvial deposits.
The next step is erosion—the process by which solid or dissolved products are transported various distances from the weathering site and are eventually deposited elsewhere as loose, unconsolidated sediments. Erosion acts to lower the overall topography of the land surface. Flowing water is the most important transporting agent for sediments, which can result in the breakage, abrasion, chemical alteration or dissolution, and sorting by size and/or shape of rock fragments and particles. This transport occurs when the velocity of the water overcomes the downward pull of gravity, and the water moves the suspended material. Sediments can also be transported by winds (as volcanic ash or fine-grained sand), by the movement of glacial ice, and by gravity (downslope sliding)—these situations, however, are less important for the creation of alluvial gem deposits.
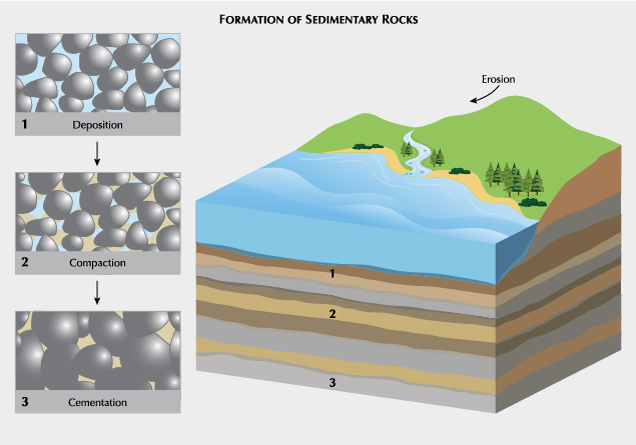
After the deposition of sediments, the final step is termed lithification. Sedimentary rocks form when layers or accumulations of clay, silt, sand, and other deposited materials are compacted and cemented by the weight of overlying rocks (figure 6). Before, during, and after lithification, sedimentary rocks undergo physical and chemical changes due to heating, compaction, and reaction with groundwater. Biological agents, including small animals or microbial bacteria, can also be important, as can chemical agents brought in by flowing water or heated hydrothermal solutions. These changes are collectively called diagenesis, and they can be described as grading into a low temperature and pressure form of metamorphism. Textural changes, including compaction and loss of pore space and trapped water, recrystallization, and the changing of fine-grained rocks into coarser ones, are all forms of diagenesis. During recrystallization, secondary minerals may precipitate in open spaces, and mineral cements such as calcite, silica, and hematite may develop—consequently, rocks become more resistant to erosion. With increasing burial conditions, sedimentary rocks become even more compacted as they are exposed to higher temperatures and pressures, which also results in the solid-state precipitation of low-grade metamorphic minerals such as chlorite. This sequence of changes by which sedimentary rocks form are described here as separate geological processes, but in actuality they merge into one another with no sharp boundaries between them.
Sandstone forms from quartz-rich sediments, whereas shale forms from those rich in clays. Limestone forms when calcite and aragonite precipitate out of water containing dissolved calcium, or it forms from the accumulation of corals and shells on the sea floor. Compaction of mixtures of pebbles or fragments results in a conglomerate. Most sedimentary rocks contain predominantly quartz, calcite, and/or various clay minerals. Fossil plants and animals are found in sedimentary rocks which have experienced conditions favorable for their long-term preservation.
SEDIMENTARY ROCK CLASSIFICATION
The nature of a sedimentary rock depends both on the source supply of the sediment as well as the depositional environment in which it formed (including continental, marine, and transitional settings involving water) (figure 7). Most sedimentary rocks can be divided into several groups based on mineral compositions and geologic formation mechanisms.
Clastic rocks are composed of rock fragments of varying sizes that are cemented together. When transported by moving agents such as water or wind, sediments often become sorted by particle size before lithification. These rocks include shale, claystone, mudstone, siltstone, sandstone, and conglomerate, with the difference between them being the increasing size of the particles from less than a millimeter to larger than a few centimeters. Most gem minerals discussed here occur in clastic rocks.
Biogenic rocks are formed by accumulations of skeletons of large or microscopic organisms or decomposed plant material. These rocks include limestone, dolostone, chert, diatomite, and coal.
Chemical sedimentary rocks are formed by inorganic constituents dissolved in and later precipitated from solution when it evaporates at normal surface temperatures. They include some limestone and dolostone (e.g., travertine), phosphorite, evaporates (such as halite), and banded iron-oxide formations.
SEDIMENTARY ROCK TEXTURES
Sedimentary rock formations often display features related to their deposition environment, such as along a river channel or coastline where sediments are deposited. These conditions can result in coarse- or fine-scale layering, banding, or bedding structures. The rocks may also exhibit cross or graded bedding, ripple marks, and mud cracks. Layering, banding, or bedding structures are due to preferential particle orientation and packing, or to the concentration of mineral particles into distinct layers. Folding and faulting of rock layers resulting from local or large-scale deformation can occur after sediment deposition and before or after rock consolidation.
Sedimentary rocks themselves display various textures resulting from the size, shape, and arrangement (packing and orientation) of the constituent particles. Particle size is of particular importance for geologic field studies because it provides information on both the conditions of transportation, sorting, and deposition of the sediment, and the geologic conditions at the deposition site. Particle shape involves the overall shape, roundness or angularity, and surface texture.
GEMS FORMED IN SEDIMENTARY ENVIRONMENTS
The formation of gem minerals in sedimentary rocks (table 1, left column; again, see figure 2) can be summarized as follows:
Opal is a hydrated form of amorphous silica produced by the deposition of spherical particles from silica-rich water flowing through cracks, pores, and voids in sedimentary rocks during hotter months as the water itself evaporates. The process is repeated over long periods of time, with the silica particles settling out over time by gravity. These tiny particles arrange themselves into regular layered structures, and they range from about 150 to 400 nanometers in diameter. Opal can produce a “play of colors” appearance by light diffraction. Both gem and non-gem opal occur widely, with the most important sedimentary deposits being in Australia (Rey, 2013; Liesegang and Milke, 2014; Hsu et al., 2015). Fire opal forms by leaching followed by deposition of silica from water moving through cavities in porous volcanic rocks. Other opal sources include Ethiopia, Hungary, Mexico, and Nevada in the United States.
Turquoise is a hydrated copper phosphate that can form in more than one way. One process begins with copper sulfide ore minerals (such as chalcopyrite or bornite) being deposited by higher-temperature hydrothermal solutions to form a mineralized rock. The turquoise forms later when meteoric waters percolate through this rock, and dissolved oxygen in the water reacts with sulfide minerals to produce soluble copper sulfates. The resulting acidic, copper-containing solution reacts with aluminum and potassium minerals in a host rock to produce turquoise that fills veins, seams, or fractures, sometimes forming nodular masses. It was among the first gem minerals to be mined, beginning more than 2000 years ago in Egypt (Ovissi et al., 2017; Ardekani et al., 2020; Shirdam et al., 2021). Important current sources include Argentina, Armenia, Australia, China, Iran, Mexico, and the United States.
Chalcedony forms in volcanic rocks that contain gas cavities, which can then be partially or completely filled with cryptocrystalline silica material that precipitates from volcanic fluids from the walls of the cavity inward. Thus, most chalcedony is not considered a gem that forms in sediments. The one exception is the green chrysoprase variety, which forms as veins, fracture fillings, and nodules in sediments due to the weathering at shallow depths of nickel-bearing serpentinite rocks that are rich in iron and magnesium (Shigley et al., 2009; Sachanbiński et al., 2023). Chalcedony occurrences are found in many localities.
Malachite and azurite are copper carbonate hydroxides that precipitate from hydrothermal fluids in fractures or underground spaces as secondary gems, followed by surface weathering and oxidation of a copper ore body. The two mineral aggregations form botryoidal, reniform, fibrous, or stalagmitic masses, with azurite sometimes also occurring as crystals. Malachite can display internal banding patterns. Both minerals have been used since antiquity as mineral pigments for decorative purposes, and both are mined by open pit and from underground workings. The major current source of malachite is the Democratic Republic of the Congo, while the less common azurite comes from various localities (Cook, 2001; Kampunzu et al., 2009; El Desouky et al., 2010; De Putter et al., 2010; Papineau, 2020).
Rhodochrosite is a less common manganese carbonate mineral that is found as distinct crystals or massive fracture- or vein-fillings associated with some ore deposits. Larger transparent crystals are sometimes faceted as gems. In a few localities, the mineral forms from dripping water which creates stalactites and stalagmites (Marquez-Zavalia and Craig, 2022).
Amber is not a mineral but an organic fossilized resin that was exuded as a sticky substance from the bark of certain trees. Pieces of resin that dropped to the ground became covered by soil. When the trees fell, the resin-containing logs were carried by rivers and tides to coastal regions where they were buried by sediments. Due to the compression pressures and elevated temperatures from the overlying sediments, the pieces of resin were compacted and transformed over geologic time, first to copal and then to amber. While long used as a gem material, amber is important for the science of paleontology since it preserves a wide variety of animal and plant matter (Grimaldi, 2003; Penny, 2010; Ross, 2010). Amber is widely distributed in rocks of Cretaceous age or younger. Historically, the southern coast of the Baltic Sea extending from Poland to Latvia has been the world’s leading source of this material. Myanmar and the Dominican Republic are other current amber sources (Wolfe et al., 2009; Weitschat and Wichard, 2010; Leelawatanasuk et al., 2013; Sun et al., 2015; Zhang et al., 2020).
While being described here as “forming” in sediments, the occurrence of these gem minerals is not directly the result of sedimentation. Instead, it involved physical and chemical changes associated with diagenesis and possibly the action of circulating hydrothermal solutions to introduce the necessary components for gem mineral formation.
GEMS FOUND IN, BUT NOT FORMED IN, SEDIMENTARY DEPOSITS
The second category of gems occurring in sedimentary environments are those that formed originally in igneous and metamorphic rocks (again, see figures 3 and 4). After these host rocks weathered, their abrasion-resistant gems were transported and are found deposited along current and former stream and river channels, either in loose or more compacted sediments. These gem minerals were formed elsewhere and were transported and concentrated by moving water and other geological processes. A placer deposit is a natural concentration of heavy minerals in secondary sediments caused by the effect of gravity on moving particles.
Alluvial or placer mineral deposits have been mined since ancient times. Because they occur at the earth’s surface, they were likely one of the earliest known types of economic ore deposit. They could be worked using simple traditional tools, and the deposits would provide an almost immediate economic reward to the miners. They continue to be significant sources of valuable metals such as gold and platinum (Ibrahim et al., 2020; Nikiforova, 2021; Cabri et al., 2022; Torvela et al., 2022).
Placer deposits form as the result of the concentrations of “heavy minerals” in secondary sediments (Patyk-Kara, 2002; Garnett and Bassett, 2005; Stanaway, 2012; Nikiforova, 2021). These deposits are masses of unconsolidated or semi-consolidated clastic sediments, formed by surface weathering and erosion of primary rocks and subsequently transported by gravity, water, wind, or ice from their original source. The heavy gem minerals must be hard, durable, resistant to weathering and breakage during water transportation, have a high specific gravity (>2.58), and possess chemical stability to be hosted in placer deposits (Joshi et al., 2021).
During water transport, fractured or other poor-quality mineral crystals are often broken up and removed, so the final alluvial deposits often contain better-quality gem material. In some instances, the gem fragments being transported become water-worn or rounded by mechanical abrasion. All of these factors can result in economically valuable mineral concentrations. Such deposits are among the most important sources of a variety of gems including diamond, corundum, spinel, garnet, and quartz, as well as nephrite and jadeite jade (table 1, right column). Such deposits often occur along current or former river channels in unconsolidated or poorly consolidated sediments. In some cases, loose gem diamonds have been carried along by glaciers, which transport but do not sort or separate the placer minerals.
TYPES OF PLACER GEM DEPOSITS
Placer deposits are classified by Dill (2018) based on the geological factors such as weathering, erosion, and local surface topography that created them (again, see figure 6).
Retained Deposits. Eluvial placer deposits are not transported and are located near the point of original gem formation in igneous or metamorphic host rocks. They are found on weathered hillslopes where lighter material has been weathered and/or carried away, but they are often not economically significant enough to support large-scale gem mining.
Transient Deposits. Colluvial deposits result from short-distance transport of material by gravity action downslope from the original gem host rock. These deposits can form at the base of steep hillsides, for example.
Alluvial deposits involve longer-distance transport mainly by water. The most significant alluvial deposits are located along bends in rivers, natural hollows, breaks in the slope of hillsides, or at the base of an escarpment or other natural barrier, and involve short- or long-distance transport of minerals by moving water. Because the transport ability varies approximately as the square of the velocity, the speed of the moving water plays an important role in deposit formation and the travel distance from the original source of the placer minerals. Thus, when the water velocity decreases, heavy minerals are deposited more quickly than the light ones. Placers can form whenever and wherever the water velocity remains below that required to transport the gems further, including bends in meandering streams, at the base of waterfalls, behind obstacles, and along eroded or hollowed-out depressions at the base of riverbeds. Over time, stream flow can remove larger and lighter minerals thereby concentrating the heavier minerals. During transport by moving water, mineral crystals or rock fragments can become abraded and waterworn.
Terminal Deposits. Beach or delta deposits form along the edge of water bodies by wave action (such as sand deposits). Continent shelf deposits occur further offshore in shallow coastal environments. Examples of the latter include diamond deposits along the southern coast of South Africa and Namibia.
Each of these three types of placer gem deposits can reside long enough to be termed a “paleoplacer,” or an ancient sediment deposit that has been buried and/or lithified to rock. Examples include the extensive Witwatersrand in Southern Africa, and the Roraima Supergroup in northern South America, which are both known to host significant amounts of diamonds and gold, and the extensive sapphire and colored stone alluvial deposits at Ilakaka, Madagascar. It should also be mentioned that in many places there are no longer any geological signs of the ancient river systems that deposited the gem gravels.
The mining of gems from natural caves is another type of occurrence that does not fit into the above classification, with the best example being the recovery of ruby and spinel from caves found in marbles. In this case, caves act as a trap for gem minerals weathered out of the marbles during karstic processes forming the caves, as well as gem-bearing sediments that may be introduced from surface-reaching openings in the cave systems.
EXAMPLES OF IMPORTANT ALLUVIAL GEM DEPOSITS
Montepuez Ruby Deposit in Mozambique. The regional geology of the countries located along the east and southeast portions of Africa is dominated by the rocks of the East African orogeny. Ruby deposits are hosted in the Montepuez metamorphic complex, consisting of Mesoproterozoic to Neoproterozoic granitic to amphibolitic orthogneisses (Boyd et al., 2010). Rubies are mined from both the host metamorphic rocks and in predominantly eluvial and colluvial type deposits derived from them. In this area, the primary ruby deposits are curiously easier to mine than secondary deposits because the metamorphic rocks have weathered predominantly to clays (Vertriest and Saeseaw, 2019).

Ilakaka Sapphire Deposit in Madagascar. Secondary deposits of sapphire and other gem minerals in the Ilakaka region include extensive buried paleoplacers of the Triassic Isalo sandstones (figure 8). These alluvial deposits have concentrated highly productive gem-bearing, poorly consolidated terraces that contain cobble- to pebble-sized rounded lithic fragments of laterite, sandstone, quartzite, and schist. They are exploited using shafts extending down about 20 m, and sometimes in open pits which target potholes or meanders in current or former rivers (Rakontondrazafy et al., 2008).

Gem Occurrences in Sri Lanka. Sri Lanka is underlain by Archean metamorphic to Proterozoic migmatites and granitoid rocks. This assemblage is overlain by loosely consolidated alluvial sediments which have accumulated over millions of years. Concentrated deposits of many types of colored gemstones derived from the basement rocks occur in sediments in various areas of the central and southern portions of the island (Dissanayake and Rupasinghe, 1993; Gunatilaka, 2007) (figure 9).
Historical Diamond Deposits in Brazil. Alluvial diamonds in Brazil are recovered from rivers and streams or in nearby unconsolidated sediments. The most productive of regions in Brazil have been the states of Minas Gerais, Bahia, and Mato Grosso. These deposits are part of a complex network of alluvial, colluvial, and eluvial deposits which vary in time and space. Compositionally, deposits are a mix of clay to sand-sized sediments, containing rounded to angular pebbles and cobbles from the surrounding bedrock including kimberlites and diamondiferous conglomerates (Farrar, 2009; Svisero et al., 2017).
Alluvial Diamond Deposits along the Vaal and Orange Rivers, and Related Continental Shelf Marine Deposits along the Atlantic Coast of Southern Africa. Diamonds from restricted kimberlite sources in the interior of Southern Africa were transported westward so that they are found along the Vaal and Orange River channels as well as underwater along an extended length of the continental shelf (Maree, 1987; Jacob et al., 1999; Moore and Moore, 2004; Wilson et al., 2006). The coastal diamond deposits of Namibia and South Africa are host to excellent gem-quality diamonds because most of the inferior stones would have been lost due to comminution during transport (Sutherland, 1982; Gurney et al., 1991; Corbett and Burrell, 2001; Bluck et al., 2005; Nakashole et al., 2018; Phillips et al., 2018; Jacob and Grobbelaar, 2019).
Sapphire Deposits in Montana. Sapphires are mined from secondary deposits in Montana at three locales: Rock Creek, the Missouri River, and Dry Cottonwood Creek (Zwaan et al., 2015; Hsu et al., 2017; Palke et al., 2023). These deposits are all related to Cenozoic volcanism, although the volcanic source rocks have not been clearly identified in all cases. The Rock Creek locality appears to be an eluvial or a colluvial deposit possibly related to rhyolitic volcanism and related ash or mudflows. Along the Missouri River, sapphires are found concentrated in gravel bars along the river banks. There is a large mining operation at Rock Creek as well as smaller artisanal operations along the Missouri River, all utilizing heavy machinery to enhance the volume processing of gravels and sapphire recovery.
Amber Deposits along the Baltic Sea Coastline. Baltic amber occurs in late Eocene to Oligocene glauconite-rich sediments (approximately 23–48 Ma in age) along the Baltic Sea coastline extending from Poland, Kaliningrad, Lithuania, and Latvia (Kasiński and Kramarska, 2008; Standke, 2008). Detailed study of the flora preserved in Baltic amber suggests a warm and temperate climate in the past. Glacial activity coincident with a marine transgression deposited amber into Pleistocene glacial tills, which are continuously eroded and deposited unto the Baltic Sea coastline (Weitschat and Wichard, 2010; Sadowski et al., 2017). Since ancient times, amber has been recovered by picking it up along the beaches (especially after storms), or by using nets and other tools to obtain it from shallow water.
LOCATING ALLUVIAL GEM DEPOSITS
Finding an alluvial gem deposit is no small task, and such occurrences are often just the result of a serendipitous discovery by an individual spotting a gem crystal fragment lying loose in the dirt along a path or exposed in a rock outcrop. Such an event often results in many local people swarming the area and digging up the ground hoping to “strike it rich.” Historic examples include the gold rushes in California in 1848 (Kelley, 1954; Eifler, 2016; Mountford and Tuffnell, 2018; Baumgardner, 2020), in Australia in 1851 (Mudd, 2007), and more recently the extensive alluvial gem deposits found in the Ilakaka region of Madagascar in the mid-1990s (figure 10; see Guerin and Moreau, 2000).

Alluvial gem deposit formation is due collectively to geological, geomorphological, and sedimentological processes. Ideally, one tries to first establish whether the geological conditions in the area would allow for the formation of certain gemstones in original host rocks (such as the presence of pegmatites which can host beryl, tourmaline, and topaz). The geomorphology of a prospective secondary location must also be conducive to collecting and storing gem crystals over geologic time through “structural traps” such as valleys, scours, dike margins, fractures, large changes in elevation, and river splays onto a floodplain. Rivers and streams must have enough water velocity and volume to move gemstone-bearing sediments into structural traps where the reduced velocity causes the gem crystals to settle out. Similarly, they settle out along the inner bends of river meanders where the water velocity is also reduced. Geologic time is also another important consideration. Alluvial sediments are constantly in flux, so the most productive alluvial deposits are those that have had enough time to remove fine clays and sands and those that have also been buried to protect them from further erosion.
MINING ALLUVIAL GEM DEPOSITS
As described above, alluvial deposits of gems and other valuable heavy minerals vary widely in their geologic occurrence, extent, and accessibility. The host sediments can also vary in their degree of compaction from unconsolidated to more cemented sedimentary rocks. In some cases, the gemstones themselves are not contained within any sediments, but they lie loose along the bottoms of bodies of water. All these factors influence the mining techniques and types of equipment used for mineral recovery (Griffith, 2013).

Both placer gems and industrial minerals are often recovered by artisanal miners (both men and women) working in the informal sector. Alluvial deposits are prospective because they typically have higher quality gem crystals, since over time inferior stones tend to be mostly destroyed by comminution, the geologic process where large particles are reduced to a smaller size. In addition, the deposits are often easier to mine with simple hand tools because the sediments are exposed near the surface, they are only partly consolidated, and the loose gems can often be recovered by washing and/or panning (figure 11).

Artisanal miners usually target alluvial gem deposits that are typically impractical in economic terms for mining companies. A few placer deposits are exploited on a larger scale by companies using more modern mining equipment and techniques (figure 12). Among these are diamond deposits on the Atlantic continental shelf and beaches of South Africa and Namibia, the ruby deposits near Montepuez in Mozambique, and the deposits of volcanic sapphire in sediments near Chanthanaburi in Thailand.
SUMMARY
Sedimentary rocks host important deposits of numerous gem minerals that either formed or have been found in these rocks. This edition of Colored Stones Unearthed summarizes the characteristic features of sedimentary rocks and describes the sedimentary geological environments where gem minerals can become concentrated. Although often limited in lateral extent, surface exposures of secondary gem deposits can often be worked with simple tools, so they are exploited by artisanal miners in many parts of the world.
A final comment is necessary. The previous two installments of this column covered gems found in igneous and in metamorphic rocks. The present entry on gems in sedimentary rocks completes the main categories of rocks exposed at the earth’s surface as would be discussed in any geology textbook. The three installments present these subjects as separate rock categories, when geological field observations sometimes produce a less clear picture. Since the environments of mineral formation among these three rock types lack distinct and accepted boundary conditions of temperature, pressure, time, and chemical composition, some surface rocks display evidence of having formed under conditions along the borders between rock categories. As mentioned earlier, for example, sediments being heated and compacted by burial undergo the process of diagenesis, whose conditions with even deeper burial can evolve into metamorphism. Therefore, mineral and gem formation can sometimes take place more along a continuum of geological conditions than might be inferred by the organization of the installments of this series.