Fluorescence Characteristics of Blue Amber from the Dominican Republic, Mexico, and Myanmar
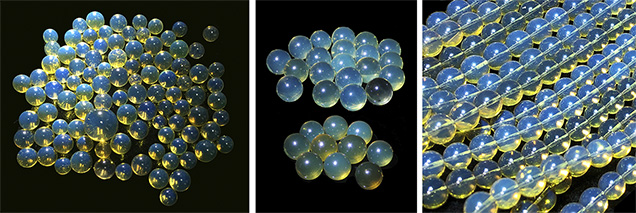
ABSTRACT
Amber shows various luminescence phenomena under UV illumination. Amber with very strong blue fluorescence under sunlight or D65 illumination can appear blue to the unaided eye when placed on a black background. Amber showing this effect is highly sought after in the Chinese gem market and referred to as “blue amber.” Almost all commercial blue amber is mined from the Dominican Republic, Mexico, and Myanmar. In this study, we collected and characterized the appearance, gemological properties, UV-visible light absorption spectra, and 3D fluorescence spectra of blue amber from these three producing areas. The results show that blue amber from the Dominican Republic generally emits much stronger fluorescence than the other two origins, with narrow triple-peak emissions near 450, 474, and 508 nm. Mexican and Burmese excitation-emission maps present several distinct emission and excitation wavelengths. Additionally, distinctive UV/visible/near-infrared absorptions at 412 and 441 nm appeared only in blue amber from the Dominican Republic. The combination of appearance, UV-Vis-NIR spectroscopy, and excitation-emission mapping may assist in the separation of blue amber from these three localities.
INTRODUCTION
Amber is widely distributed around the world. It is of interest not merely as an important natural fossil resin witnessing the history of the earth and providing direct evidence for paleobiologists, but also as a widely loved organic gemstone. Transparent yellow amber from the Dominican Republic, Mexico, and Myanmar displaying a blue or greenish blue glow when viewed on a black background in normal sunlight is called “blue amber” in the Chinese gem trade. This paper focuses on amber displaying this characteristic and will refer to this material as blue amber. In the Guangzhou and Shenzhen amber trading centers, Dominican blue amber can be sold for up to thousands of Chinese RMB (around several hundred U.S. dollars) per gram, while Mexican material fetches only several hundred RMB (around several tens of U.S. dollars) per gram—even though they have a similar appearance.
Bellani et al. (2005) first described blue amber and studied the fluorescence emission, excitation, and optical absorption spectra of Dominican blue and non-blue (including yellow and red varieties) amber. They found that multiple emission fluorescence peaks at 449 nm, 476 nm, and 505 nm occurred in this blue amber, while only a broad fluorescence band was observed in non-blue amber. They further proposed that perylene (a polycyclic aromatic hydrocarbon, C20H12) was the fluorophore for Dominican blue amber by comparing the emission spectra and fluorescence lifetime with the fluorescence spectra documented in the handbook by Berlman (1971). Liu et al. (2014) found blue amber’s fluorescence to be confined to the surface, and further illustrated that a black background could enhance the visibility of the fluorescence by reducing the amount of scattered light that reaches the eye. Chekryzhov et al. (2014) and Bechtel et al. (2016) discussed the luminescence properties and the hydrocarbon composition of blue amber from the Russian Far East, but they did not give a possible cause of the blue fluorescence. Recently, Jiang et al. (2017) analyzed the fluorescence emission wavelengths and optimal excitation wavelengths, aiming to distinguish Dominican from Mexican and Burmese blue amber. Additionally, the fluorescence properties of Baltic Sea and Ukrainian amber have been described using conventional and synchronous fluorescence spectrometers (Matuszewska and Czaja, 2002; Mysiura et al., 2017).
The main purpose of this work is to fully characterize blue amber from the Dominican Republic, Mexico, and Myanmar, as well as to compare their gemological properties, UV-Vis-NIR spectra, and 3D fluorescence spectra. To make the following discussion more applicable to practical identification workflow, we classify these amber samples according to their apparent fluorescence colors (blue, greenish blue, and non-blue) and then discuss the difference in EEM (also known as 3D fluorescence spectra, excitation-emission matrix, or excitation-emission mapping) from different origins in each category.
SAMPLES AND METHODS
Samples. For this study, we contacted merchants in the Chinese gem trade who dealt mainly with specific amber species and could provide samples with reliable origins as well as current market information. Figure 1 shows commercial blue amber beads from three sources. We tested a total of 247 amber specimens (including beads, fragments, and jewelry pieces) reportedly from the Dominican Republic (109), Mexico (47), and Myanmar (91).
Gemological Testing. Standard gemological and spectroscopic testing were all performed at the Gemmological Institute of China University of Geosciences (Wuhan). Specific gravity (SG) values were determined hydrostatically using a Sartorius BT 224 S balance. Refractive indices (RI) were measured with a GR-6 refractometer produced by Wuhan Xueyuan Jewelry Technology Co. Ltd. Reaction to long-wave ultraviolet light (LWUV: 365 nm) and short-wave ultraviolet light (SWUV: 254 nm) was observed using a standard handheld gemological 4W UV light source (mercury bulb), also made by Wuhan Xueyuan Jewelry Technology Co. Ltd. Samples and inclusions were observed and photographed using a Leica M205 A microscope and a Nikon D810 camera. For each bead, two sets of images were captured—one on a white background and the other on a black background, to show the difference in appearance.
UV-Vis-NIR Spectroscopy. UV/visible/near-infrared absorption spectra were measured in transmission mode with a PerkinElmer Lambda 650 S spectrophotometer. Spectral scans were collected from 250 to 800 nm with a speed of 266.75 nm/min and a data interval of 1.00 nm. The detector response was set at 0.2 s. To successfully perform transmission spectroscopy, the samples needed to be prepared as slices; all slices were less than 0.5 mm in thickness. It is not possible to perform this type of destructive testing on samples intended for jewelry.
Fluorescence Spectroscopy. All fluorescence spectra were recorded at room temperature using a Jasco FP-8500 fluorescence spectrometer (see box A). 3D fluorescence spectral data (i.e., EEM) was collected with a 2000 nm/min scan speed. The excitation wavelengths varied from 220 to 500 nm, with a step size of 5 nm and an excitation bandwidth of 5 nm. The emission spectra were collected with a starting wavelength 10 nm longer than the excitation wavelength and up to 750 nm, with the bandwidth set to 5 nm and a data interval of 1 nm. The beam size was fixed with a 3 mm diameter aperture. In order to keep the illuminated area constant, the sample was placed in a fixed position in a sample holder with a 2 mm diameter opening (see figure A-2). Each EEM, made up of 57 individual emission scans, usually took about 20 minutes to collect. The final EEM spectra appeared similar to a contour map with color coding to indicate the strength of the fluorescence emission peaks or bands under various excitation wavelengths (see box B).
After identifying the main emission peaks, specific excitation wavelengths were chosen and finer-resolution emission scans were performed to obtain more detailed spectra for these specific excitation wavelengths. These scans were conducted with the excitation bandwidth set to 2.5 nm, emission bandwidth 1 nm, and the data interval 1 nm.
Data Collection. All data were recorded using Spectra Manager II software from Jasco. The same software package was used to construct the EEMs from 57 rows and 511 columns of data; higher-resolution emission spectra were drawn as typical two-dimensional plots using plotting software.
RESULTS
Standard Gemological Properties. All samples were colorless to yellow to orange under a standard D65 light when placed on a white background, but the Burmese samples tended to have a richer yellow color. However, when viewed on a black background under a standard D65 light source, three types of color appearance were easily identified—blue fluorescence (B-type), greenish blue fluorescence (GB-type), and fluorescence that was too weak to see and thus labeled as non-blue amber (NB-type) (such as the examples seen in figure 2). Liu et al. (2014) attributed the color appearance change from yellow to blue to the superposition of the reflected light with its fluorescence. Most blue amber in the market has an evenly distributed blue or greenish blue color across the entire surface. Among all 247 samples we studied, we found that those from the Dominican Republic and Mexico included both B- and GB-types, while Myanmar had only B-type. NB-type amber occurred in all three sources.
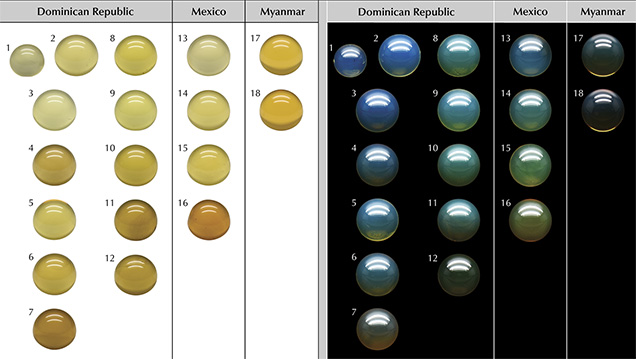
Table 1 summarizes the samples by color appearance and the results of standard gemological testing. The RI values by spot measurement were all 1.54, while the SG values ranged from 1.032 to 1.051 for material from the Dominican Republic, 1.030 to 1.048 for material from Mexico, and 1.024 to 1.042 for material from Myanmar. When observed under the microscope, the samples were quite transparent, with a few tiny internal features. The fluorescence response was typically LWUV > SWUV, with or without the phosphorescence. Under LWUV illumination, Dominican and Mexican blue amber showed blue fluorescence, while the Burmese samples sometimes displayed a violetish blue fluorescence.
UV-Vis-NIR Spectroscopy. We collected in total 178 spectra from 81 Dominican amber samples (including 76 B- and GB-type, and 5 NB-type), 44 Mexican amber samples, and 53 Burmese amber samples. Typical UV-Vis-NIR absorption curves for blue amber are shown in figure 3. We noticed that the absorption edges of samples from all three origins were similarly located at about 400 nm. A tailing absorption from 400 to 500 nm indicates that when mixed-wavelength sunlight strikes blue amber, wavelengths shorter than 400 nm cannot penetrate deeply, while light between 400 and 500 nm may be able to penetrate a little deeper, resulting in a yellowish bodycolor. In Dominican blue amber samples, two distinctive absorption peaks occurred at 412 and 441 nm as well, thus further limiting the 400–450 nm light penetration for Dominican samples. These two peaks are distinctive features for blue amber from the Dominican Republic and were not detected in material from Mexico or Myanmar. However, both the size (or thickness) and the color of samples impact the detection results, so this test has its limitations in real-world gem testing.
Fluorescence Spectroscopy. A brief introduction to 3D fluorescence spectra (EEMs) and relevant terms and symbols are provided in box B.
After collecting 3D fluorescence spectra of all samples, totaling 247 EEM spectra, we found that the fluorescence intensity varied in different samples, while the excitation-emission maps had similar features for samples of the same type from the same origin. To describe blue amber fluorescence features in detail, we selected 18 samples (numbered and shown in figure 2), which had typical EEMs representing the various groups of all blue amber in this study. They included 11 pieces of B-type with different fluorescence intensity (samples 1–7 from the Dominican Republic, 13–14 from Mexico, and 17–18 from Myanmar); six beads of GB-type with different fluorescence intensity (samples 8–11 from the Dominican Republic and 15–16 from Mexico); and one non-blue amber (sample 12) from the Dominican Republic.
Fluorescence of B-Type Amber. Figure 4 compares the 3D fluorescence spectra and significant emission and excitation curves of B-type amber from the Dominican Republic, Mexico, and Myanmar.
From the EEMs of all Dominican B-type samples (74 specimens, figure 4A), we found that the fluorescence emission consisted of three peaks that were rather stable when excited by different excitation wavelengths. Figure 4B shows a typical emission spectrum using λex = 415 nm; three main peaks at wavelengths of λem = 450, 474, and 508 nm are clearly visible. These three fluorescence peaks are in good agreement with those reported by Bellani et al. (2005), Chekryzhov et al. (2014), Liu et al. (2014), and Jiang et al. (2017). The observed fluorescence intensity Iλem=450 nm is generally about the same as Iλem=474 nm, though it is sometimes a bit stronger. The strongest fluorescence emission can be excited by λex = 415 and 441 nm, but the strongest λem is observed using 441 nm excitation (figure 4C). Additionally, λem can only be excited using λex between 350 and 460 nm, thus explaining the somewhat inert reaction to SWUV.
Compared with their Dominican counterparts, all Mexican and Burmese B-type samples tended to have weaker Iλem, as indicated by the lower maximum values for fluorescence intensity in their EEM spectra, as in figures 4A, 4D, and 4G. Their emission patterns were also rather different.
In Mexican B-type samples, shown in figure 4, D–F, when excited using λex = 441 and 415 nm, one can see similar triple-peak patterns made up of 451, 476, and 514 nm emissions. Upon switching to a shorter excitation wavelength of λex = 400 nm, two different emission bands appeared near 438 and 456 nm (figure 4E). Additionally, the most efficient excitation region in these samples was from 320 to 475 nm, which is rather far from the typical SWUV (254 nm radiation found in a handheld UV light source), thus resulting in the very weak to inert fluorescence appearance under SWUV.
In Burmese B-type (seen in figure 4, G–I), a three-emission pattern similar to that of the Dominican B-type was observed in the longer excitation wavelengths (380–450 nm), indicated in figure 4G as the area outlined by a rectangle. The more interesting feature in these samples is in the shorter excitation wavelengths. Two unique emissions were observed: An additional emission peak at about λem = 425 nm (with a violet color) is at its maximum when excited with λex = 375 nm (figure 4I); another λem at approximately 347 nm (in the ultraviolet region) was seen in a wide range of excitation wavelengths, including 240 nm (figure 4H) and from 270 to 330 nm. Again, these spectral features explain the observed weak response to handheld SWUV illumination, especially when compared to the response to LWUV illumination at 365 nm.
Fluorescence of GB-Type Amber. Figure 5 compares the EEM spectra and emission and excitation curves of GB-type amber from the Dominican Republic and Mexico.
In all Dominican GB-type amber, the EEM patterns are similar to those in B-type (for example, figures 4A and 5A). The major differences include: (1) Iλem=474 nm is much higher than Iλem=450 nm, regardless of the excitation wavelengths, and (2) a longer-wavelength emission tailing toward the green region (indicated by the rectangular area in figure 5A). This tailing varies as the excitation wavelength changes. This emission tailing enhances the green fluorescence, which explains the greener fluorescence appearance in the GB-type amber. Additionally, (3) another excitation extremum at λex = 461 nm occurs in excitation curves (figure 5C).
As for Mexican GB-type, the EEMs of samples 15 and 16 (figure 5, D-G) display a broad emission range from the near-ultraviolet to the greenish yellow region (350 to 600 nm), which can be excited with a wide range of excitation wavelengths from 275 to 500 nm. The strongest emission in this material is around 435 nm and/or 464 nm.
Fluorescence of NB-Type Amber. Figure 6 displays the EEM pattern of Dominican NB-type amber. The highest Iλem of this amber is only 600, versus the much higher emission counts for B- and GB-type amber, showing maximum values of 8000 to 6500, respectively. This observation indicates that non-blue amber from the Dominican Republic has a much lower fluorescence strength than the other two types from the same locality.
The NB-type amber shows a weak broad emission band in the blue to yellow region (410 to 600 nm) with the highest peak at λem = 463 nm, best excited by 400 nm light. This is similar to Mexican GB-type amber, but the latter has stronger emission (maximum values of fluorescence intensity are 1300–1700 vs. 600 in Dominican NB-type; see figures 5D and 5F and figure 6A). Due to the wide excitation range and stronger emissions of Mexican GB-type, fluorescence can still be observed visually with sunlight, whereas it cannot in Dominican NB-type (sample 12).
DISCUSSION
In our UV-Vis-NIR absorption spectra (figure 3), the Dominican blue amber showed additional absorption peaks at 412 and 441 nm, which is consistent with the optimal excitation wavelengths for its blue fluorescence. This indicates that Dominican blue amber is very efficient at absorbing these two wavelengths and more likely to generate stronger fluorescence than its Mexican and Burmese counterparts.
When conducting fluorescence measurements, one needs to consider the effects of self-absorption and secondary fluorescence, which can cause errors in fluorescence spectral analysis. These two effects are related—self-absorption occurs when a sample under testing absorbs its own primary fluorescence (Birks, 1974; Lakowicz, 2006) and subsequently emits secondary fluorescence that is either similar to or different from the primary fluorescence. Such absorption may decrease the total amount of fluorescence or reduce a specific peak intensity measured by the instrument, and the secondary fluorescence may produce additional emission peaks or bands. These two effects are more often encountered in a typical fluorescence spectral measurement when the sample is a liquid and loaded in a standard cuvette with 1 cm optical path length. Fortunately, our experiment geometry (as described in box A) can minimize the possibility of self-absorption and secondary fluorescence. Liu et al. (2014) clearly indicated that the fluorescence only occurs on the amber’s surface, not deep inside. In addition, our UV-Vis-NIR absorption spectra (figure 3) also showed that any light with wavelength <400 nm will be greatly absorbed and cannot penetrate the amber sample. Thus, the excitation light source is highly unlikely to travel deep into the amber sample to cause self-absorption and secondary fluorescence. Our geometry also ensures that the sample size will not directly influence the amount of fluorescence emission measured.
When we observe amber’s fluorescence with the unaided eye, our eyes and brain are extremely sensitive to the fluorescence intensity variations, which allows us to classify the fluorescence response as inert, weak, medium, strong, or very strong. In the current experiment, by strictly controlling the EEM measurement parameters, we achieved a rough quantification of the fluorescence intensity—the intensity bar of the EEM can reflect the relative strength of each sample’s fluorescence response. Nevertheless, this procedure may help us set up a future semi-quantitative fluorescence scale for very strong, strong, medium, weak, and inert responses.
Considering the similarities and differences in EEMs from different types of Dominican, Mexican, and Burmese blue amber, it seems possible that EEMs could be aid in separating geographic origin. Figure 7 shows a preliminary flowchart for this separation—by combining the appearance under D65 on a black background, the UV-Vis-NIR absorption (should such test be allowed), and the EEM fluorescence spectra. This summary is based upon the 247 samples we studied and will be further tested and expanded when more samples are available.
Determining the nature of the chemical compound that produces the different fluorescence features is an exceedingly difficult task due to the structural complicacy resulting from polymerization and the low solubility of amber in most solvents. To date, only Bellani et al. (2005) proposed a possible candidate—perylene—for the triple-peak fluorescence features in Dominican blue amber. Chekryzhov et al. (2014) and Bechtel et al. (2016) excluded perylene for Russian Far East blue amber and proposed azulene (C10H8) as an alternative agent of fluorescence but did not confirm it. Material from the Russian Far East is not commercially available and therefore was not included in this study. Nevertheless, the exact fluorescing agent still has not been successfully extracted, nor has its structure been determined.
CONCLUSIONS
In this study, blue amber refers to amber that shows significant blue fluorescence under a standard D65 light source and, when placed on a black background, this material appears blue to greenish blue. This type of amber is known to come from the Dominican Republic, Mexico, and Myanmar, and material from all three origins exhibits similar gemological properties such as bodycolor, transparency, RI, SG, and internal features. However, their LWUV responses are different—Dominican and Mexican amber mostly show blue fluorescence, while Burmese material displays a violetish blue fluorescence. Blue amber from all three origins showed a similar response to SWUV—much weaker to inert, as compared to the LWUV responses.
We separated blue amber into two types according to their appearance in sunlight or D65 illumination when viewed against a dark background. One is B-type with a blue appearance, and the other is GB-type with a greenish blue appearance. All three origins produce amber that does not show enough blue fluorescence to change its appearance in D65 light; we classified this material as NB-type.
In their EEMs, a triple-peak emission pattern always occurs in Dominican blue amber (both B-type and GB-type). B-type and GB-type Dominican blue amber differ in that the GB-type amber has an additional green fluorescence component (~ 500 nm emission) and an additional 461 nm excitation maximum.
For Mexican B-type amber, the triple-peak features are merged into each other and additional emissions near 438 and 456 nm showed up when using <400 nm excitation. Mexican GB-type amber only has a broad emission feature with an emission center near 460 nm, similar to Dominican NB-type amber. However, Mexican GB-type amber has much stronger emission intensity than Dominican NB-type amber. Burmese B-type amber, in addition to displaying a triple-peak feature similar to Dominican material, has emissions in the violet-ultraviolet region, giving it a violetish appearance.
In the UV-Vis-NIR absorption spectra, in addition to the strong absorption edge just below the near-ultraviolet region (<400 nm) that blue amber from all three sources display, Dominican blue amber has two absorption peaks at 412 and 441 nm, which correspond to the most efficient excitation wavelengths in the EEM. This may explain why Dominican amber fluoresces with much more intensity. Combining the appearance, UV-Vis-NIR spectra, and EEMs, a possible workflow for discriminating blue amber from these three geographic origins is proposed (figure 7). Further study continues to identify the nature of the fluorescing agents and their relationship to the tree species that secrete the specific resins.