Geographic Origin Determination of Ruby
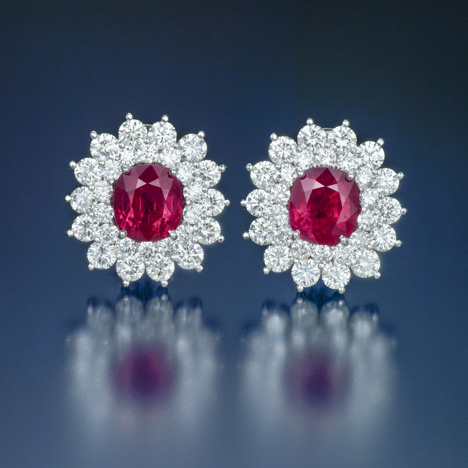
ABSTRACT
Over the last several decades, geographic origin determination for fine rubies has become increasingly important in the gem trade. In the gemological laboratory, rubies are generally broken down into two groups based on their trace element chemistry: marble-hosted (low-iron) rubies and high-iron rubies. High-iron rubies are usually a straightforward identification based on their inclusions and trace element profiles. Marble-hosted rubies can be more challenging, with some deposits showing overlap in some of their inclusion scenes. But many marble-hosted rubies, especially Burmese stones from Mogok and Mong Hsu, can be accurately identified based on their internal features and trace element profiles. This contribution will outline the methods and criteria used at GIA for geographic origin determination for ruby.
INTRODUCTION
The world ruby market has changed dramatically in modern times, especially in the last decade with the discovery and development of ruby mining in Mozambique (Chapin et al., 2015). As the gem trade has witnessed turbulent and dramatic changes in the ruby supply chain, the concept of geographic origin has become increasingly important to people buying and selling fine rubies. Often, the origin of a ruby plays an important role in the value placed on the stone, especially for exceptional quality rubies from Myanmar (figure 1). As with other colored gemstones, the ruby trade relies to a large extent on reputable gemological laboratories to make these origin determinations. In the second material-specific article of this series we delve into the world of rubies, their gemological properties, and characteristics that may aid in geographic origin determination. The following sections will detail the origin data GIA has collected for rubies and describe the methodology used in the lab to apply this data to geographic origin work.
SAMPLES AND ANALYTICAL METHODS
Rubies included in this study are predominantly from GIA’s reference collection, which was assembled over more than 10 years by GIA’s field gemology department. Stones in GIA’s reference collection were obtained by skilled gemologists from reliable sources and were collected as close to the mining source as possible (see Vertriest et al., 2019, pp. 490–511 of this issue). When necessary, the data from the reference collection were supplemented by stones from the personal collections of the authors of this study or from GIA’s museum collection. The trace element data were collected from 280 marble-hosted ruby samples and 219 high-iron samples: 65 samples from Myanmar, 93 from Vietnam, 74 from Afghanistan, 48 from Tajikistan, 34 from Cambodia, 7 from Thailand, 93 from Madagascar, and 85 from Mozambique.
Trace element chemistry was collected over the course of several years at GIA using two different laser ablation–inductively coupled plasma–mass spectrometry (LA-ICP-MS) systems. The ICP-MS used was either a Thermo Fisher X-Series II or iCAP Qc system, coupled to an Elemental Scientific Lasers NWR 213 laser ablation system with a frequency-quintupled Nd:YAG laser (213 nm wavelength with 4 ns pulse width). Ablation was carried out with 55 μm spot sizes, with fluence of 8–10 J/cm2 and repetition rates of either 15 or 20 Hz. 27Al was used as an internal standard at 529250 ppmw with custom-developed synthetic corundum used as external standards (Wang et al., 2006; Stone-Sundberg et al., 2017). Detection limits varied slightly through the course of the analyses but were generally 0.1–0.3 ppma Mg, 0.5–2.0 ppma Ti, 0.03–0.2 ppma V, 5–20 ppma Fe, and 0.03–0.07 ppma Ga. Cr is not used in the laboratory as a discriminant element, after years of testing showed it is not useful for origin. Additionally, ruby/pink sapphire calls are made based on color and not Cr concentrations. Trace element values are reported here in parts per million on an atomic basis rather than the more typical parts per million by weight unit used for trace elements in many geochemical studies. Units of ppma are the standard used in GIA laboratories for corundum, as this allows a more facile analysis of crystal chemical properties and an understanding of the color mechanisms of sapphire and ruby. Conversion factors are determined by a simple formula that can be found in table 1 of Emmett et al. (2003). The reference samples are diverse in terms of their appearance and presence/absence of silk, clouds, and otherwise included areas. Every effort was made to sample as many chemically distinct areas in heterogeneous samples as possible to ensure robust representation of silky, cloudy, and unincluded ruby trace element chemistry.
Inclusions were identified, when possible, using Raman spectroscopy with a Renishaw inVia Raman microscope system. The Raman spectra of the inclusions were excited by a Modu-Laser Stellar-REN Ar-ion laser producing highly polarized light at 514 nm and collected at a nominal resolution of 3 cm–1 in the 2000–200 cm–1 range. In many cases, the confocal capabilities of the Raman system allowed inclusions beneath the surface to be analyzed.
UV-Vis spectra were recorded with a Hitachi U-2910 spectrometer or a PerkinElmer Lambda 950 in the range of 190–1100 nm with a 1 nm spectral resolution and a scan speed of 400 nm/min. UV-Vis-NIR spectra are presented as absorption coefficient (a) in units of cm–1 where a = A × 2.303/t, with A = absorbance and t = path length in cm.
MARBLE-HOSTED AND HIGH-IRON RUBIES
As with blue sapphires, the first step in making a geographic origin determination for rubies is to make a broad division into two groups—in this case, marble-hosted rubies and so-called high-iron rubies. The first group encompasses rubies from the legendary mines in Mogok in what is now Myanmar, as well as more modern deposits in Vietnam, Tajikistan, Afghanistan, and Mong Hsu in Myanmar. These marble-hosted ruby deposits are mostly located in Southeast and Central Asia and were formed during the Himalayan orogeny. During this geological event, platform carbonates (limestones) that formed in the warm shallow sea between the Indian and Asian plates were buried and subjected to extreme temperatures and pressures when these two plates collided. Fluids and molten salts that circulated through the marbles formed in this process were responsible for mobilizing aluminum and chromium in these rocks and forming ruby (Garnier et al., 2008; Giuliani and Groat, 2019, pp. 464–489 of this issue).
On the other hand, “high-iron ruby” is a catchall term used to describe the second group of rubies, which can be distinguished by their distinctive trace element chemistry. Rubies in this group have much more diverse geological origins, ranging from basalt-related rubies such as those found in Thailand and Cambodia to more metamorphic or metasomatic rubies found in Mozambique and Madagascar. The common thread, geologically speaking, seems to be that rubies in this group probably were derived from similar geological formations, which was most likely (ultra)mafic or basic intrusive igneous rocks (Mercier et al., 1999; Smith et al., 2016; Fanka and Sutthirat, 2018; Sutthirat et al., 2018; Palke et al., 2018). It was these iron-rich rocks that imparted their characteristic trace element profiles on the rubies (see Giuliani and Groat, 2019, pp. 464–489 of this issue, for more detailed discussion of the geology of these deposits).
Unfortunately, there is no spectroscopic test available to easily distinguish between these two groups of rubies as there is for metamorphic and magmatic blue sapphires. In many cases, a glance through the microscope can easily make this separation, as typical inclusion scenes tend to be different between the two groups. Intensity of fluorescence can also be an indicator: The iron in the “high-iron” group tends to inhibit fluorescence, and typically these rubies do not fluoresce as strongly as the marble-hosted rubies. In order to improve the accuracy of a geographic origin conclusion, however, trace element chemistry is important to consider as well. The marble-hosted rubies generally have iron concentrations below 200 ppma, with very few stones above 400 ppma, while iron contents in the high-iron rubies are mostly above 400 ppma. Assessing all the available data allows easy separation of rubies from these two groups in most cases, simplifying geographic origin determination by significantly reducing the number of potential locations. Those stones falling in between these two groups, with an iron content between 200 and 400 ppma, need to be considered very carefully, as several origins are possible in these cases.
GEOGRAPHIC ORIGIN DETERMINATION: MARBLE-HOSTED RUBY INCLUSIONS
In many cases, the geographic origin of marble-hosted rubies is difficult to identify in the laboratory. Historically, things would have been simpler. For hundreds if not thousands of years, the mining town of Mogok in Burma (now Myanmar) was practically the sole source of this group of rubies (Themelis, 2008). The Afghan ruby mines at Jegdalek have had some production for several hundred years, but the output has never matched Mogok’s in terms of quantity or quality (Garnier et al., 2008; Hughes et al., 2017). However, Mogok rubies started to see much more competition starting in the twentieth century with new finds of marble-hosted rubies in Vietnam, Tajikistan, and another deposit in Myanmar at Mong Hsu. Because all of these rubies were formed in the same geological event under similar geological conditions, their inclusion scenes and especially their trace element chemistry can sometimes be similar to those from the legendary deposit in Mogok.
For many stones coming through GIA’s lab, inclusion scenes and trace element chemistry are enough to accurately determine a geographic origin. This is usually the case for Burmese rubies from Mogok and Mong Hsu, which often have typical inclusions. However, the reality of the situation is that in some cases a stone may not contain a diagnostic inclusion scene and the trace element chemistry may be ambiguous. In these situations, an “inconclusive” origin determination is the only appropriate answer. In the following sections we will lay out the exact criteria currently employed by GIA for making geographic origin determinations for marble-hosted rubies. Additional information about these rubies can be found in the following references: Kane et al. (1991), Brown (1992), Hughes (1994); Peretti et al. (1995), Smith (1998), Bowersox et al. (2000), Schwarz and Schmetzer (2001), Long et al. (2004a,b,c), Gübelin and Koivula (2008), Sorokina et al. (2015), and Hughes et al. (2017).
Typical Inclusion Scenes. Inclusion scenes often supply the bulk of the evidence in ascertaining geographic origin for marble-hosted rubies. Unfortunately, there are not any “diagnostic” mineral inclusions for rubies. All marble-hosted rubies can contain inclusions of calcite, apatite, and other common solid minerals. More often, the defining inclusion scenes are composed of characteristic patterns of silk, milky clouds, and patterned clouds. In the following sections we will offer examples of typical inclusion scenes for marble-hosted rubies from Myanmar, Vietnam, Afghanistan, and Tajikistan.
The Internal World of Burmese Rubies. Rubies come from two separate deposits in Myanmar: the historic Mogok region and the more recent Mong Hsu deposit. In general, Mogok has produced more important, finer-quality rubies (figures 2 and 3) while Mong Hsu has mostly supplied commercial-grade material. In the following sections, the inclusion scenes of Mogok and Mong Hsu rubies will be considered independently.
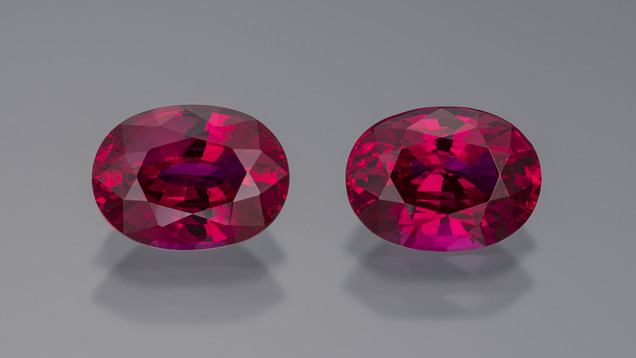
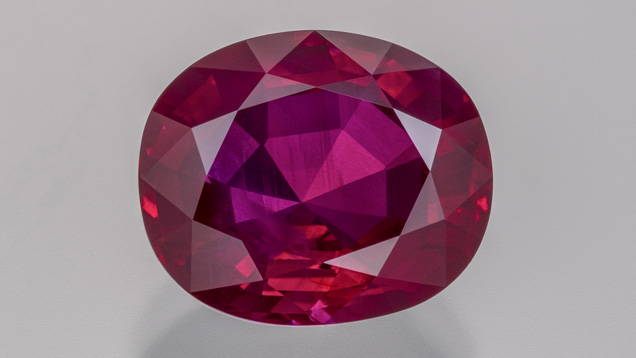
Several photomicrographs depicting typical inclusion scenes in Mogok rubies are shown in figures 4–9. The silk in Mogok rubies often occurs as nested concentrations of fine, long to short rutile needles (figure 4). These nests of silk often occur near the center of the stone, sometimes accompanied by inclusions of calcite and other minerals (figure 5). Silk in Mogok rubies often occurs as short reflective rutile needles with a flattened aspect, possibly exhibiting an arrowhead shape due to twinning of the rutile (figures 6–8). However, longer silk is also common in Mogok rubies (figures 4 and 5). Note that unlike many rubies from other deposits, Mogok rubies usually do not require heat treatment to optimize their color. This means that silk in these stones generally stays intact. While heat treatment of blue sapphires tends to destroy evidence of origin in the form of silk patterns, this is rarely the case for the silk seen in Mogok rubies. Another inclusion scene suggestive of a Mogok origin is distinctive roiled graining, which is likely caused by turbulent and rapid crystal growth when the rubies formed (figure 9). Distinct red and colorless zoning is often associated with this graining. Note that Burmese rubies from Mogok can often be identified by characteristic inclusion scenes such as iridescent short needles or nested silk, though there may be borderline cases where the inclusions are not conclusively Mogok but might still indicate such an origin (see the “Inclusion Scenes Gone Wrong” section below).
The ruby deposits in Mong Hsu were discovered only recently, in the later part of the twentieth century. Luckily, Mong Hsu rubies tend to have characteristic inclusion scenes that allow them to be easily separated from rubies from Mogok and other marble-hosted rubies. Because dark blue zones and fractures are so common in Mong Hsu rubies in their natural state, most stones from this deposit are heat treated at high temperatures. Unheated Mong Hsu rubies are often distinguished by a dark blue core (figure 10). However, unheated material from Mong Hsu is rare. More commonly seen is a white, cloudy core produced by the heat treatment process (figure 11). Patterned clouds such as ladders and snowflake inclusions are typical of Mong Hsu rubies (figures 12 and 13). Stepped, angular graining is occasionally seen in Mong Hsu rubies as well (figure 14). More recently, flux-assisted heating of rubies has been widely used with Mong Hsu rubies, and flux-healed fractures are prevalent in much of the material on the market. Solid mineral inclusions are rare in these rubies.
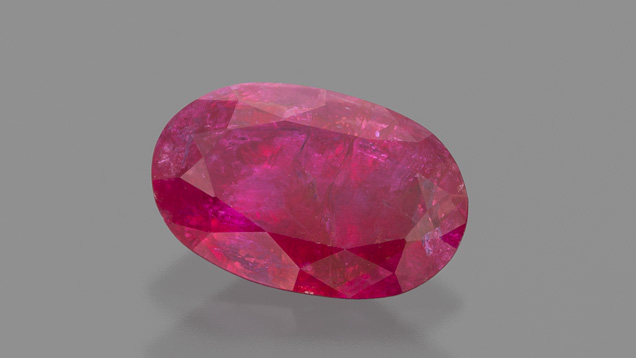
The Internal World of Vietnamese Rubies. The ruby deposits in Vietnam, discovered in the late twentieth century, have yielded fine rubies of exceptional quality (figures 15 and 16), rivaling those from the famed Mogok Valley (Kane et al., 1991; Brown, 1992; Khoi et al., 2011). As with most marble-hosted rubies, there tends to be significant overlap in their properties between various geographic localities, especially when it comes to trace element chemistry (see the “Trace Element Chemistry” section below). However, Vietnamese rubies may have distinctive inclusion scenes that help in determining their provenance. Especially characteristic is the presence of dense, angular milky banding (figures 17 and 18). Coarse, patterned clouds such as snowflake and ladder inclusions are relatively common in Vietnamese rubies and tend to be larger than those found in Mong Hsu rubies (figure 18). Coarse, oriented silk is also seen in some Vietnamese rubies, sometimes resembling the silk in Mogok rubies, although their inclusion scenes can usually be distinguished by the experienced gemologist. Vietnamese rubies often have blue color zoning that can be somewhat irregular in nature (figure 19), as well as roiled growth patterns in some stones. Heat treatment is often used in an attempt to remove blue color zoning in these rubies, but this is often at a low enough temperature that a stone’s silk may not be dramatically affected. Rounded mineral crystals can also be seen in Vietnamese rubies, although these are not considered diagnostic (figure 20).
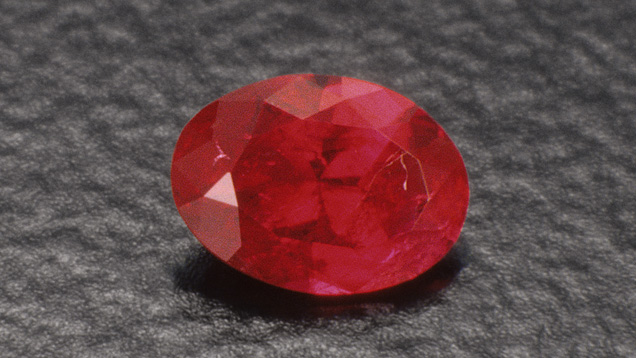
The Internal World of Afghan Rubies. The ruby mines near Jegdalek in Afghanistan are considered one of the classic sources from the ancient world, believed to date back around 800 years (Bowersox et al., 2000). However, supplies have always been limited, especially for rubies with high clarity that could compete with fine rubies from Myanmar. The dominant inclusions in much of the material available are fractures and fingerprints. Unfortunately, high-quality material with sufficient clarity has been difficult to obtain, which has limited thorough study of the characteristic inclusions in this material. The following discussion will detail the information gathered so far by GIA’s field gemology program. Afghan rubies can sometimes be distinguished by characteristic fine rutile needles (figure 21) and fine particulate clouds, often found in angular or hexagonal patterns (figure 22), which are distinct from the milky clouds found in Vietnamese rubies or the coarser and needle-like silk seen in Mogok rubies. Planar milky clouds can also be found in Afghan rubies (figure 23), but they tend to be fainter than those seen in Vietnamese rubies and are not easily observed without fiber-optic illumination. Stringer-type inclusions formed of small dusty particulates are sometimes seen in Afghan rubies (figure 24), but they are also found in rubies from Tajikistan. Fluid-filled fingerprints are also seen in Afghan rubies and (figure 25).
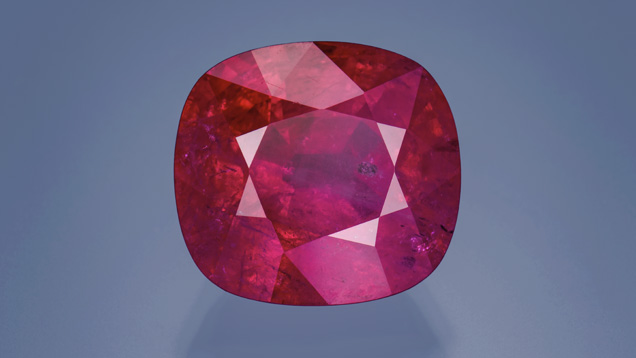
The Internal World of Tajik Rubies. Tajik rubies are another relative newcomer to the world ruby mining scene (figure 26). Discovered in the 1970s by Soviet geologists, only small quantities have been produced from the Snezhnoe deposit in southern Tajikistan near the Chinese border (Smith et al., 1998; Sorokina et al., 2015). Further, much of what is produced has been heavily included, lower-quality material. Nonetheless, some very fine rubies are known to have been sourced from Snezhnoe. Tajik rubies can be difficult to separate from other marble-hosted deposits, partly due to the relative lack of diagnostic inclusions. Much like Afghan rubies, the dominant inclusions are fractures and healed fissures (fingerprints). Also notable, and indicative of a Tajik origin, are so-called rosettes or haloes surrounding small crystal inclusions (figure 27). Blue color zoning is also frequently seen in Tajik rubies (figure 28). It can be distinguished from similar features observed in Vietnamese rubies by the angular and very planar nature of the blue zones, in contrast to the more irregular blue zoning in Vietnamese rubies. Tourmaline crystal inclusions seem to be a diagnostic mineral inclusion in Tajik rubies (figure 29). These tourmaline inclusions are often found in small, irregular clusters that include mica and graphite inclusions. Also observed are fine, dusty distributions of particles (figure 30) and sometimes stringer inclusions composed of fine particulate matter (figure 31). However, similar features are seen in Afghan rubies.
Inclusion Scenes Gone Wrong. With geographic origin determinations for marble-hosted rubies, one encounters the same problem as with metamorphic blue sapphires: The gems from these deposits were mostly formed in the same geological orogenic event, from very similar geological formations, and likely at similar conditions of pressure, temperature, and chemistry. For this reason, there tends to be significant overlap in their gemological properties, namely inclusions and trace element chemistry. Care needs to be taken, especially with Vietnamese and Mogok rubies, as occasionally their inclusion scenes overlap. Importantly, Vietnamese rubies can sometimes be found with patterns of rutile silk that might give the initial impression of a Mogok origin. Inclusion scenes suggestive of Mogok rubies, composed of short to long well-defined needles of rutile silk (sometimes densely packed and intergrown) can occasionally be found in rubies from Vietnam (figures 32 and 33). Additionally, the roiled graining considered characteristic of Mogok rubies can also occasionally be found in Vietnamese rubies (figure 34). Observation of dense milky clouds, if present, might help to resolve overlap and indicate a Vietnamese origin. Other potentially conflicting inclusion scenes include the presence of patterned clouds in Tajik and Afghan rubies that might give an initial impression of a Vietnamese ruby (figures 35 and 36). Whenever possible, additional gemological evidence should be sought out to corroborate an origin conclusion. For instance, milky clouds might suggest a Vietnamese origin or arrowhead rutile needles might indicate a Mogok origin. However, it bears emphasizing that the gemological world is not perfect. Not every faceted stone will contain inclusion scenes that are diagnostic of a particular locality. Moreover, many stones do not have inclusion scenes that are even suggestive of one origin over another. A marble-hosted ruby may lack milky or patterned clouds and contain only coarse rutile silk that is not particularly nested and dense, and not iridescent. In this case, the inclusion scene could give the impression of either a Burmese or Vietnamese origin, offering scant evidence to indicate one origin over another. In these cases, the only clear choice is to offer an “inconclusive” origin determination. Unfortunately, there will always be stones for which laboratories will be obligated to issue “inconclusive” calls due to the lack of any diagnostic piece of evidence pointing toward a specific conclusion.
High-Iron Ruby Inclusions. If trace element analysis places a stone in the high-iron ruby group, the geographic origin determination process follows a different path from marble-hosted rubies, with a distinct set of possible provenances. These are rubies from Thailand and Cambodia, Madagascar, Mozambique, and smaller deposits in Tanzania, Kenya, and Greenland. Fortunately, “high-iron ruby” is more of a broad catchall term with relatively loose implications about geological conditions of formation. For instance, high-iron rubies from Mozambique and Madagascar formed during high-pressure and high-temperature regional metamorphism, while those mined near the Thai/Cambodian border are basalt-related and, in some ways, more geologically akin to classic magmatic blue sapphires. The common thread seems to be the rubies’ derivation from similar host rocks. While there are many open questions about their exact geological genesis, high-iron rubies are generally considered to have been derived from what were originally basic or (ultra)mafic igneous rocks. Nonetheless, variations in the specific geological circumstances surrounding ruby genesis have produced differences in their gemological properties, allowing for straightforward origin determination in most cases.
Rubies from most of the high-iron ruby deposits (Mozambique, Madagascar, and Thailand/Cambodia) can often be separated based only on inclusions, but even if microscopic observations fail to produce a conclusion, trace element chemistry can almost always provide the final piece of evidence. In the following section we will describe typical inclusion scenes for the major high-iron ruby deposits. Additional information can be obtained from the following references (Schwarz and Schmetzer, 2001; Gübelin and Koivula, 2008; Pardieu et al., 2012; Hughes et al., 2015, 2017; Vertriest and Saeseaw, 2019).

The Internal World of Thai/Cambodian Rubies. Rubies were mined in the ancient kingdom of Siam, along the present-day Thai/Cambodian border, for hundreds of years (figure 37). Until relatively recently, these Siamese rubies were the only serious alternative to those mined in Mogok. Back then it would have been straightforward to separate them from Mogok rubies, and even today the Thai/Cambodian rubies are easy to identify. Note, however, that rubies from the Chanthaburi-Trat area in Thailand are virtually identical to those found just across the border in Pailin, Cambodia, as the mining area is essentially one single deposit that straddles this geographic border (Sangsawong et al., 2017). One of the most striking microscopic features of Thai/Cambodian rubies is their lack of any rutile silk. The most prominent features are negative crystals, melt inclusions, and voids with partially healed decrepitation haloes that show geometric patterns and iridescent colors when using fiber-optic illumination (figures 38 and 39). These haloes are all oriented in the same crystallographic direction and are very transparent when light is not being reflected off them. When the correct angle is found in the microscope, they all light up at once, sometimes with colorful displays of iridescence. Also seen are similar reflective platelet-type inclusions, which are ostensibly not centered around a larger parent inclusion. Solid mineral inclusions are relatively uncommon but include diopside and rarely garnet or pyrrhotite (figure 40). Twinning is prevalent in Thai/Cambodian rubies, and the intersection tubules between twinned sectors are often filled with diaspore or other aluminum (oxy)hydroxides (figure 41). Note that Thai/Cambodian stones can almost always be identified in the lab by their signature inclusion scenes.
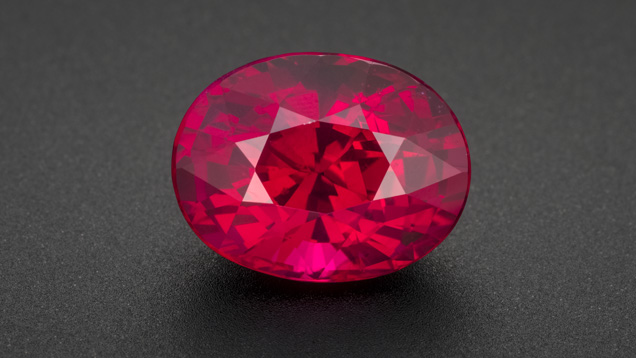
The Internal World of Mozambique Rubies. The rise of Mozambique in the early twenty-first century completely upended the global ruby market. Never before had such vast quantities of high-quality ruby (figure 42) been so freely available to the gem and jewelry industry. This could have resulted in a significant problem for the gemological laboratories had the Mozambique ruby not been fairly straightforward to identify (Vertriest and Saeseaw, 2019). In many cases, a Mozambique origin can be concluded based on observations of inclusion scenes, but additional evidence is always sought in the form of trace element chemistry, which almost always closes the case (see the “High-Iron Ruby Trace Element Chemistry” section below). Common mineral inclusions in Mozambique rubies are grayish green amphiboles (figure 43) and hexagonal mica booklets with stress fracture fringes (figure 44). Sulfide and spinel inclusions are also observed (figure 45). Mozambique rubies also can contain angular particulate clouds (figure 44, left), but they are more frequently identified by their characteristic fields of reflective, platelet-like particles (figures 46 and 47). Like the haloes seen in Thai/Cambodia rubies, these platelets are all oriented in the same crystallographic direction and may be difficult to see until that direction is found. Then they will all become visible at once. As with the rest of the high-iron rubies, Mozambique rubies can generally be identified in the lab by their inclusion scenes and trace element chemistry.
The Internal World of Madagascar Rubies. Madagascar rubies are the other East African newcomer in the ruby market (Schwarz and Schmetzer, 2001; Pardieu et al., 2012; Hughes et al., 2015). While they have never reached the commercial importance of Mozambique and much of the production is commercial grade, some exceptional ruby has been derived from Madagascar. Gemologically and geologically, Madagascar’s rubies are most similar to those from Mozambique. Muscovite inclusions are occasionally encountered in rubies from Madagascar (figure 48) as well as amphibole, which may generally have a distinctive morphology with longer, more prismatic rod-like crystals (figure 49). However, the more common and characteristic inclusion in rubies from Madagascar is zircon, especially widely distributed clusters of small, rounded zircons (figures 50 and 51). Blocky and prismatic orange, brown, or black rutile inclusions can also be encountered and suggest a Madagascar provenance (figure 52). Particle clouds may be encountered as well, often composed of short needles and fine particulates, but sometimes mixed with long needles (figure 53). The particle clouds usually show a well-defined hexagonal arrangement, and there is the possibility of confusion with similar features seen in Mozambique rubies. Occasionally Madagascar rubies also host angular milky clouds, a feature not characteristic of the other major high-iron ruby deposits. Madagascar rubies are generally easy to identify in the laboratory based on their inclusion scenes and trace element chemistry.
Trace Element Chemistry of Marble-Hosted Ruby. When attempting to use trace elements to discern a marble-hosted ruby’s origin, one has the same problem encountered with metamorphic blue sapphires: The very similar geological conditions of formation for these rubies creates very little deviation in their trace element profiles. When the compositional data are plotted together for the major economic deposits, separation can seem almost hopeless. The selective plotting method developed for blue sapphires (see Palke et al., 2019, pp. 536–579 of this issue) is also applied to origin determinations for marble-hosted rubies using the same suite of trace elements (Mg, Ti, V, Fe, and Ga). The ranges of trace element profiles seen in marble-hosted rubies can be found in table 1. By filtering out reference data with dissimilar trace element profiles and comparing an unknown stone only against reference stones that match the unknown, the trace element plots are greatly simplified, making it easier to interpret the data. In some cases, the selective plotting method is very useful for marble-hosted rubies and can provide evidence of a stone’s provenance that would not have been obvious otherwise (figure 54). (Note that all trace element data are produced from LA-ICP-MS; see Groat et al., 2019, pp. 512–535 of this issue.) But as with metamorphic blue sapphires, in some cases there is simply too much overlap in the trace element data and no amount of statistical analysis or variation in plotting the data will help (figure 55). Unfortunately, this is the limitation of geographic origin determination imposed by the forces of nature and geology that created these fine stones. An “inconclusive” call is always warranted if the gemological evidence does not produce any clear indication of origin. Note that for the sake of clarity the plots shown here include only the major marble-hosted ruby deposits, while some smaller deposits (especially from East Africa) are not included. In laboratory practice, the trace element data for these deposits can be considered as possibilities if there is other corroborating evidence.
Trace Element Chemistry of High-Iron Ruby. Stones in the high-iron ruby group are much easier to separate than marble-hosted rubies by using multiple lines of evidence. Even in cases where microscopy alone cannot determine a ruby’s provenance, which is fairly uncommon, the addition of trace element analysis almost always results in an accurate origin conclusion. The full range of trace element profiles for high-iron rubies can be found in table 2. Several trace element plots are shown in figure 56, illustrating how the use of only a few trace elements can almost always separate high-iron rubies from the major mining areas. (Note that all trace element data are produced from LA-ICP-MS; see Groat et al., 2019, pp. 512–535 of this issue.) The selective plotting method used for blue sapphires and marble-hosted rubies at GIA (see Palke et al., 2019, pp. 536–579 of this issue) is sometimes used for high-iron rubies as well, but in most cases it is not necessary. Note that for the sake of clarity, these plots show only the major high-iron ruby deposits, while some smaller deposits such as those in Tanzania, Kenya, and Greenland are not included. In laboratory practice, the trace element data for these deposits can be considered as possibilities if there is other corroborating evidence. Additional information about these deposits can be found in Thirangoon (2009), Smith et al. (2016), and Hughes et al. (2017).
CONCLUSIONS
The twentieth and twenty-first centuries have seen dramatic changes in the world ruby market. The rapid increase in ruby sources across the world has largely benefited the gem-consuming public by making these precious stones more accessible over a wide range of price points. However, as the gem industry has come to incorporate geographic origin in determining the value of these stones (e.g., the Burmese ruby and sapphire in figure 57), this diversification of ruby sources has proven to be a great challenge for gemological laboratories. Through 10 years of efforts, GIA’s field gemology and research departments have amassed world-class collections of rubies from the major world sources. The data collected on these reference stones has been crucial in allowing GIA to make origin determinations with the utmost confidence and integrity (even when the origin call is necessarily “inconclusive”). Nonetheless, the data and methods used at GIA for making ruby origin determinations, as described in this article, demonstrate the oftentimes precarious nature of origin determination in gemological laboratories.
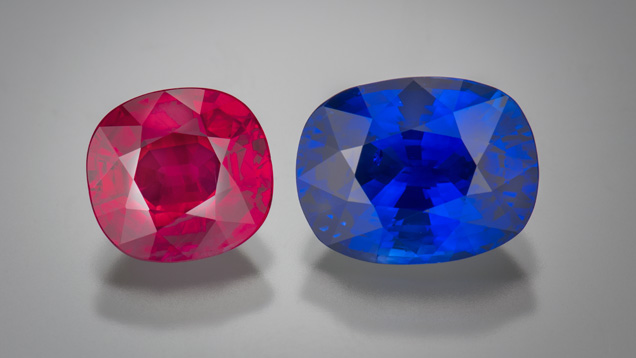
Many stones present characteristic inclusion scenes that lead to a straightforward origin decision. This is often the case with rubies from Thailand, Mozambique, Madagascar, and rubies from some of the marble-hosted deposits, especially those from Myanmar (figure 58). Rubies from the other marble-hosted deposits such as Vietnam, Afghanistan, and Tajikistan are generally more challenging. Trace element chemistry can be useful, especially for so-called high-iron rubies. Unfortunately, and especially for marble-hosted rubies, trace element chemistry can often be ambiguous.
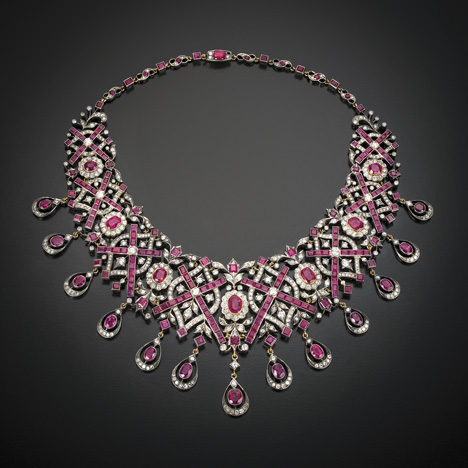
The use of the “selective plotting” method described herein may help in making sense of this trace element data, but it is hardly a cure-all. In cases where the inclusion scenes and trace element evidence are ambiguous, the only possible determination is an “inconclusive” origin. Future research and field gemology efforts will focus on advancing our understanding of ruby origin determination and refining the methods used in the laboratory at GIA. However, it is almost certain that there will always be rubies with a lack of characteristic origin data to support an origin conclusion. While further work may help us reduce the number of “inconclusive” calls, the overlap in gemological properties for many of our reference stones makes it unlikely that this number will ever reach zero.