Geographic Origin Determination of Emerald
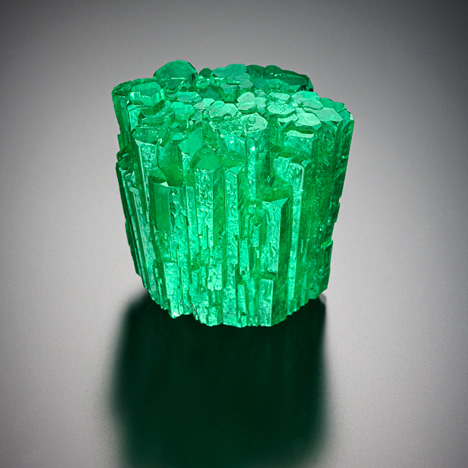
Abstract
The gem trade has grown to rely on gemological laboratories to provide origin determination services for emeralds and other fine colored stones. In the laboratory, this is mostly accomplished by careful observations of inclusion characteristics, spectroscopic analysis, and trace element profile measurements by laser ablation–inductively coupled plasma–mass spectrometry (LA-ICP-MS). Inclusions and spectroscopy can often separate Colombian emeralds from other sources (although there is some overlap between Colombian, Afghan, and Chinese [Davdar] emeralds). For non-Colombian emeralds, trace element analysis by LA-ICP-MS is needed in addition to information from the stone’s inclusions. The relative chemical diversity of emeralds from worldwide deposits allows confidence in origin determination in most cases. This contribution outlines the methods and criteria used at GIA for geographic origin determination for emerald.
INTRODUCTION
When the Spanish conquistadors first brought Colombian emeralds (figures 1 and 2) onto the international market, they became a global sensation in their day. Emeralds from Central Asia and Egypt were known at the time, but the world had likely never seen emeralds of such high quality and size. Traders soon developed distribution channels that brought the Colombian material all the way from the royal courts in Europe to the powerful Moguls of India (Giuliani et al., 2000).
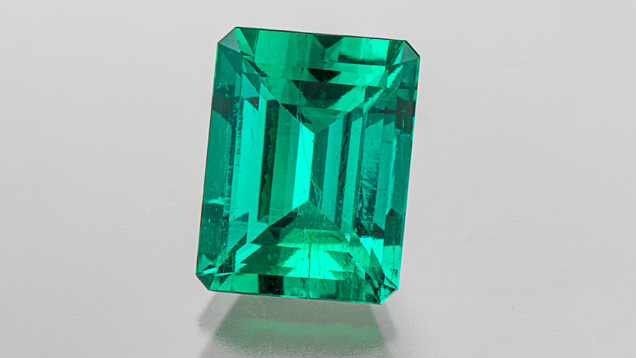
The nineteenth and twentieth centuries saw all of this change as new mines sprang up around the world to challenge the famed Colombian emeralds. Most notable in terms of high-quality production were Brazil, Russia, and Zambia, but smaller deposits have been uncovered in Madagascar and Ethiopia and elsewhere. As the market has evolved alongside these developments, geographic origin has come to be an important factor for fine-quality emeralds. The demand for emerald origin determination was initially driven by the proliferation of sources. However, this expansion and diversification of emerald sources has also complicated origin determination. As the number of sources grows, so does the overlap in their characteristics. The following sections will detail the specific criteria used in the laboratory at GIA to make geographic origin conclusions for emeralds, as well as potential areas of overlap and how these are dealt with.
SAMPLES AND ANALYTICAL METHODS
Emeralds included in this study are dominantly from GIA’s reference collection, which was assembled over more than a decade by GIA’s field gemology department. Stones in GIA’s reference collection were obtained from reliable sources and collected as close to the mining source as possible; see Vertriest et al. (2019), pp. 490–511 of this issue. When necessary, the data from the reference collection was supplemented by stones gathered by the authors of this study outside of the field gemology program or borrowed from GIA’s museum collection. The trace element data was obtained from 298 samples total, with 36 from Zambia (25 from Kafubu and 11 from Musakashi), 34 from Colombia, 36 from Afghanistan, 22 from Madagascar, 64 from Russia, 49 from Brazil, 32 from Ethiopia, and 25 from China (Davdar). Emeralds from Madagascar are much less frequently encountered in the lab. Therefore, these deposits are included in the trace element section here, but their inclusions are not described for the sake of brevity and clarity. More information about specific mines within these countries can be found in Vertriest et al. (2019),pp. 490–511of this issue.
Trace element chemistry was collected at GIA over the course of several years using two different LA-ICP-MS systems. The ICP-MS was either a Thermo Fisher X-Series II or iCAP Qc system, coupled to an Elemental Scientific Lasers NWR 213 laser ablation system with a frequency-quintupled Nd:YAG laser (213 nm wavelength with 4 ns pulse width). Ablation was carried out with 55 μm spot sizes with fluence of 8–10 J/cm2 and repetition rates of either 7, 10, or 20 Hz.29Si was used as an internal standard at 313500 ppm using NIST 610 and 612 as external standards. Repeat analyses on samples from single locations over time have verified the consistency of the analyses with these various setups. Accuracy is estimated to be within 10–20% for most elements analyzed, based on comparisons with electron microprobe data on a small selection of samples.
Inclusions were identified, when possible, using Raman spectroscopy with a Renishaw inVia Raman microscope system. The Raman spectra of the inclusions were excited by a Stellar-REN Modu Ar-ion laser producing highly polarized light at 514 nm and collected at a nominal resolution of 3 cm–1 in the 2000–200 cm–1 range. In many cases, the confocal capabilities of the Raman system allowed inclusions beneath the surface to be analyzed.
UV-Vis spectra were recorded with a Hitachi U-2910 spectrometer or a PerkinElmer Lambda 950 in the range of 190–1100 nm with a 1 nm spectral resolution and a scan speed of 400 nm/min. When possible, polarized spectra of oriented samples were collected to obtain o- and e-ray absorption spectra. UV-Vis-NIR spectra are presented either as relative absorbance with no units or absorption coefficient (a) in units of cm–1 where a= A × 2.303/t, with A = absorbance and t = path length in cm.
RESULTS
UV-Vis-NIR Spectroscopy in Emerald Origin Determination. As with rubies and sapphires, emerald’s geographic origin determination starts with a coarse separation into one of two groups based on their geological conditions of formation: hydrothermal/metamorphic and schist-hosted/magmatic-related (the Type II tectonic metamorphic-related and Type I tectonic magmatic-related groups from Giuliani et al., 2019; Giuliani and Groat, 2019, pp. 464–489 of this issue). This distinction also roughly corresponds to a separation between Colombian emeralds (or those emeralds that might have Colombian-like features) and essentially everything else. The hydrothermal/ metamorphic group contains emeralds from Colombia, Afghanistan, and China (Davdar), while the schist-hosted/magmatic group includes emeralds from Zambia (Kafubu), Russia, Ethiopia, and Brazil, among others. In many cases, this separation can be made based on gemological properties. Hydrothermal/metamorphic emeralds often have jagged multiphase inclusions, whereas schist-hosted emeralds generally have blocky or irregular multiphase inclusions. The difference in appearance is likely related to variations in the way the fluids were originally entrapped as well as post-entrapment healing and evolution of the inclusions while the emeralds were held at high temperature and pressure before being exhumed to the earth’s surface (e.g., Giuliani et al., 2019).
But advanced analytical techniques are often more reliable than relying solely on inclusions. Schist-hosted emeralds generally have much higher Fe content than hydrothermal/metamorphic emeralds. This chemical distinction is most easily seen using UV-Vis-NIR spectroscopy. Representative UV-Vis-NIR o-ray spectra of hydrothermal/metamorphic emeralds are shown in figure 3 (top). The most prominent features in this group are the Cr3+ broad absorption bands at about 430 and 600 nm and the sharp Cr3+ band at around 683 nm. A typical Colombian UV-Vis-NIR spectrum is characterized by the absence of any absorption bands in the near-IR region at wavelengths greater than 700 nm (bottom spectrum in figure 3, top). Other members of the hydrothermal/ metamorphic emerald group (i.e., Davdar, China, and Afghanistan), which generally have higher concentrations of Fe, usually show a noticeable to significant absorption band at 810 nm caused by Fe2+, possibly enhanced by nearby Fe3+. This feature has traditionally been used to separate Colombian emeralds from those from all other deposits (e.g., Saeseaw et al., 2014). However, as shown in figure 3 (top), there is some overlap between some Colombian emeralds and other members of the hydrothermal/metamorphic group. On average, Colombian emeralds have lower Fe content than stones from any other deposit. However, the few stones at the higher range of Fe content for Colombian emeralds have a noticeable Fe2+ absorption band at 810 nm. Similarly, while Afghan emeralds tend to have higher Fe content than most Colombian stones, at their lower Fe concentration range some stones may show only a slight Fe-related absorption at 810 nm, which could be mistaken for a classic Colombian emerald spectrum. Great care must be taken in interpreting UV-Vis-NIR spectra to identify possible Colombian emeralds, and multiple lines of evidence, including trace element chemistry, must be used to accurately ascertain a Colombian origin.
Due to their much higher Fe content, schist-hosted emeralds are easy to separate from Colombian and other hydrothermal/metamorphic stones based on their o-ray UV-Vis-NIR absorption spectra (figure 3, bottom). The most obvious distinction is the significantly increased intensity of the Fe-related 810 nm absorption band in schist-hosted emeralds. Additionally, these higher-iron emeralds also tend to have a more prominent Fe3+ absorption band at 372 nm than the hydrothermal/metamorphic emeralds. As with the hydrothermal/metamorphic group, making geographic origin determinations for schist-hosted emeralds requires the use of multiple lines of evidence including UV-Vis-NIR analysis, trace element chemistry, and microscopic observation of a stone’s inclusions.
Microscopic Internal Inclusions. Emeralds, even top-quality material, tend to be fairly heavily included. Fluid inclusions, partially healed fissures, needles, and/or solid mineral crystals are frequently encountered. Inclusions are an extremely important tool for geographic origin determination as well as separating natural and synthetic emeralds. However, there are several areas of overlap. Emeralds from some deposits may have inclusion scenes that resemble those seen in other important deposits. Some stones may lack any diagnostic inclusions, making it impossible to provide an origin opinion if there is no definitive trace element data. Gemologists often classify emerald into two broad groups based on fluid inclusions: jagged or blocky. This also corresponds roughly to the hydrothermal/metamorphic and schist-hosted classification presented above. Most fluid inclusions in emerald are multiphase, but it is often difficult to observe all the distinct phases in an inclusion using a gemological microscope. Jagged fluid inclusions usually appear to be composed of a liquid, a gas, and one or more solid phases. Sometimes there is even more than one liquid phase present as well. Regardless, when there are clearly observed solid, liquid, and gas phases present, these are typically referred to as three-phase inclusions. Blocky fluid inclusions often appear to be composed of only two phases, a liquid and a gas. However, there is often a solid phase present in these inclusions, which is hard to observe due to similarity in refractive index between the solid and fluid. Sometimes the use of cross-polarized light reveals hidden solid crystals in these fluid inclusions. Additional information about the internal features of emeralds from major world deposits can be found in the following references: Giuliani et al. (1993, 2019), Vapnik et al. (2006), Groat et al. (2008), Marshall et al. (2012), Zwaan et al. (2012), Saeseaw et al. (2014), and Vertriest and Wongrawang (2018).
Geographic Origin Determination of Hydrothermal/Metamorphic Emeralds. Colombian emeralds are the most economically important from the hydrothermal/metamorphic type of deposit, and jagged fluid inclusions are their hallmark features. Such fluid inclusions are typically elongate, with jagged sawtooth edges. Unfortunately, emeralds from other hydrothermal/metamorphic deposits such as Afghanistan and China may have similar jagged fluid inclusions. Among the mineral inclusions found in these emeralds are calcite, pyrite with various morphologies, albite, and carbonate minerals. This section will document the internal features of emeralds from Colombia, Afghanistan, and China. Emeralds from a minor deposit at Musakashi in Zambia will also be mentioned briefly at the end of this section. Further information on the inclusion scenes in emeralds from these deposits can be found in Bowersox et al. (1991), Bosshart (1991), and Saeseaw et al. (2014).
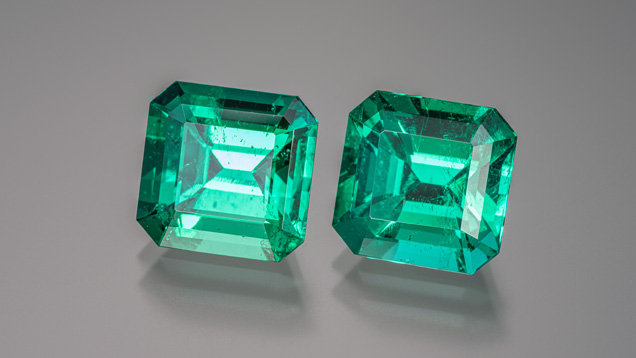
The Internal World of Colombian Emeralds. Colombian emeralds (figure 4) have long been held in high esteem for their rich green color and for the history and legends associated with these stones. The most readily identifiable feature of Colombian emeralds are classic three-phase jagged fluid inclusions. These contain a gas bubble and a cubic crystal (typically halite and/or sylvite) and generally range in size from 100 μm to 1 mm (figures 5 and 6). However, as mentioned above, jagged fluid inclusions can be seen in emeralds from other deposits such as Afghanistan and China (see also Saeseaw et al., 2014). Large jagged fluid inclusions (greater than about 500 μm) are unique to Colombia and can be taken as diagnostic evidence of origin. However, when only smaller jagged fluid inclusions are observed, care must be taken to ascertain a stone’s origin and additional supporting evidence should be sought out. Gota de aceite is a growth feature sometimes seen in Colombian emeralds that can be used as a strong indication of origin (figure 7). Similar growth features are sometimes observed in emeralds from other sources such as Zambia, but it is by far most common in emeralds from Colombia. This growth feature’s name is Spanish for “drop of oil,” in reference to the roiled and turbid appearance it lends. Gota de aceite grows parallel to the basal faces, often in planes that do not extend throughout the stone. Common solid inclusions in Colombian emeralds are carbonates, pyrite (figure 8), quartz, feldspar, and small black particles from the surrounding black shales (Giuliani et al., 2019; Giuliani and Groat, 2019, pp. 464–489 of this issue). However, most of these minerals are also found in other emerald deposits. Similar to Kashmir sapphires, Colombian emeralds also have one very rare mineral inclusion that has never been seen in emeralds from other deposits: parisite (Gübelin and Koivula, 2008). When observed, it can be considered a diagnostic indicator of Colombian origin; unfortunately, this inclusion is not frequently encountered.
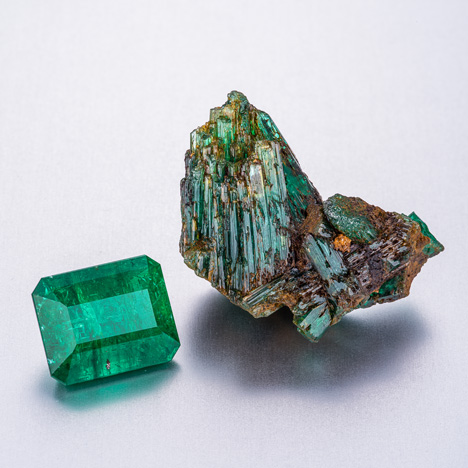
The Internal World of Afghan Emeralds. While Afghan emeralds have never held the market share of their Colombian brethren, many fine stones have been produced from the mines in the Panjshir Valley (figure 9). As for their internal characteristics, multiphase fluid inclusions are the most common inclusion in Afghan emeralds. They often have an elongate, needle-like shape and host several daughter minerals (figures 10 and 11), which can distinguish them from Colombian and Chinese emeralds. Daughter minerals in fluid inclusions in emeralds are often assumed to be halite when they have a cubic habit; however, when there are several daughter minerals, as in the inclusions in Afghan emeralds, their exact identity is often hard to determine, even with the use of confocal Raman spectroscopy. The typical inclusion scene in these emeralds from the Panjshir Valley consists of small jagged fluid inclusions (figures 12 and 13), similar to those seen in Colombian emeralds, and scarce crystalline inclusions. Solid inclusions, when present, include pyrite, limonite, beryl, carbonate minerals, and feldspar.
The Internal World of Chinese Emeralds. The deposit at Davdar, China, was discovered late in the twentieth century. The geology of Davdar is not well understood, but it is reported that this deposit shares some similarities with other metamorphic deposits (Giuliani et al., 2019). Fluid inclusions in these emeralds have a jagged shape and are composed of a rounded gas bubble and a cubic crystal (figures 14–17). They are often small and can resemble those seen in emeralds from Colombia and Afghanistan. While there is likely very little production from the Chinese mines reaching the market, their potential for overlap with Colombian emeralds makes it important to be aware of their identifying characteristics to avoid potential problems in origin determination.
Inclusion Scenes Gone Wrong. Many Colombian emeralds are easily identified by large jagged inclusions (figure 6) or gota de aceitegrowth features (figure 7). Multiphase inclusions containing numerous daughter crystals (figure 10) are considered conclusive evidence of an Afghan origin. However, it can be complicated in smaller or less-included stones where there may not be much information available to the microscopist. For example, the inclusion scenes of two Afghan emeralds in figures 18 and 19 showed jagged edges with a rounded gas bubble and cubic crystal(s). These inclusions could easily be misinterpreted as Colombian. Similarly, emeralds from a minor deposit in Zambia at Musakashi can also have jagged three-phase inclusions similar to those seen in Colombian emeralds, making it necessary to search for further evidence (figures 20 and 21). As with the Chinese emerald deposit at Davdar, few stones have emerged from Musakashi. Nonetheless, it is important to be aware of this potential overlap in properties. UV-Vis-NIR analysis can be helpful to separate between Colombian and Afghan, Chinese, or Musakashi emerald. Many Afghan, Chinese, and Musakashi emeralds have obvious Fe-related absorption bands distinguishing them from Colombian stones. However, there will be some overlap in the high-Fe Colombian emeralds and the low-Fe Afghan and Chinese emeralds, both of which may show minor Fe-related absorption bands. For these borderline cases, chemical analysis is needed for a conclusive result.
Geographic Origin Determination for Schist-Hosted Emeralds. Schist-hosted emerald deposits are the result of magmatic processes, including pegmatitic events. Emeralds formed in these deposits through interaction of pegmatites or other magmatic bodies with mafic, ultramafic, and/or metamorphic country rocks. This type of emerald includes important sources such as Zambia (Kafubu), Brazil, Russia, and Ethiopia. These emeralds tend to have a darker green color than Colombian, Afghan, and Chinese emeralds owing to their generally higher iron content. However, some lower-iron schist-hosted emeralds, especially those from Russia, may have lighter-toned colors. Schist-hosted emeralds often have blocky fluid inclusions that can be either two-phase, three-phase, or multiphase. The most common solid crystal inclusion is mica, which occurs in a variety of forms and colors. Mica can be formed before the emerald (i.e., protogenetic) or at the same time as the host crystal (syngenetic). Other mineral inclusions can also be found: dendritic black inclusions and quartz, carbonate minerals, talc, pyrite, emerald, chlorite, and spinel. The following sections will describe typical inclusion scenes for each origin. Note that emeralds from Madagascar would be included in the schist-hosted group. However, these emeralds are much less frequently encountered in the lab and so they are not included in the discussion of inclusion scenes. Nonetheless, origin determination of Madagascar emeralds will be considered later in the trace element chemistry section. Further information on the inclusion scenes in schist-hosted emeralds can be found in Cassedanne and Sauer (1984), Hänni et al. (1987), Zwaan et al. (2005), Saeseaw et al. (2014), Vertriest and Wongrawang (2018), and Palke et al. (2019a).
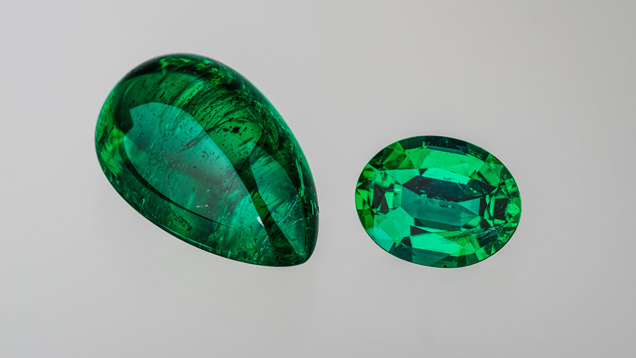
The Internal World of Zambian Emeralds from Kafubu. Zambian emeralds (figure 22) are found in the Kafubu area in the southern part of the historically important copper mining area known as the Copperbelt. Emerald mineralization occurs at the phlogopite-biotite contact zone between pegmatites and a talc-magnetite schist. Zambian emerald fluid inclusions are typically blocky (figure 23) or irregularly shaped (figure 24) multiphase inclusions. Mica is a common inclusion in Zambian emeralds from Kafubu, occurring with a brownish color and rounded shape (figure 25) or in pseudo-hexagonal green platelets (figure 26). Black platelets, or dendritic inclusions composed of oxide minerals such as magnetite, hematite, or ilmenite (figure 27), can be seen in Zambian and other schist-hosted emeralds. Elongate amphibole crystals are also observed occasionally in Zambian emeralds (figure 28). Other minerals such as apatite, pyrite, talc, barite, albite, and calcite have also been reported (Saeseaw et al., 2014).
The Internal World of Brazilian Emeralds. Emeralds have been discovered in several Brazilian localities including Carnaíba and Socotó (Bahia), Santa Terezinha (Goiás), and Itabira (Minas Gerais). This article will focus on production from Itabira, or the Belmont mine, as it is the main producer of Brazilian emerald today. Characteristic inclusions for emeralds from Belmont include blocky fluid inclusions and parallel tiny tubes described as “rain-like” (figure 29) that, if dense enough, can occasionally produce chatoyancy. The fluid inclusions typically show a blocky shape (figure 30) and may have multiple liquid/gas/solid phases apparent within (figure 31). Some irregular fluid inclusions in Brazilian emeralds may have concentric equatorial fractures (figure 32). Mica can be found as both syngenetic pseudo-hexagonal (figure 33) and protogenetic rounded brown crystals (figure 34). Other solid mineral inclusions such as quartz, magnetite, chromite spinel, calcite, or pyrite can also be observed, similar to other deposits of schist-hosted emerald.
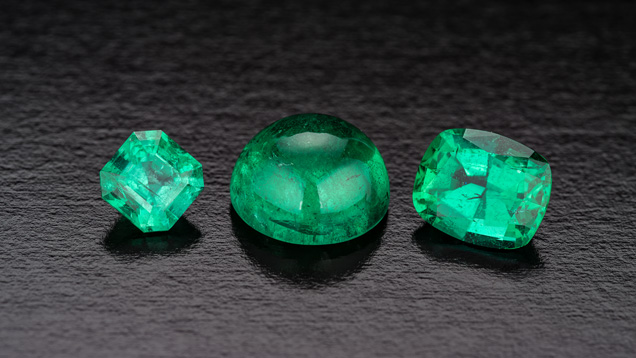
The Internal World of Ethiopian Emeralds. In late 2016, emerald was discovered in Ethiopia near the village of Shakiso (figures 35 and 36). Their fluid inclusions often reveal blocky, elongate, or irregular multiphase inclusions (figures 37–39) and nail-like growth blockage inclusions (figure 40). The blocky inclusions are very similar to those seen in emeralds from Zambia (Kafubu), Brazil, and Russia. Some fluid inclusions in Ethiopian stones contain two separate liquid phases, one gas bubble, and often solid crystals (liquid/liquid/gas) (figures 37–39). When these stones are examined in a microscope using an intense incandescent light, one of the fluids can evaporate and merge with the gas, which has been identified by Raman spectroscopy as a CO2 gas. Some inclusions may also have granular fringes (figure 39). Minute particles and iridescent thin films (figure 41) are seen occasionally and can be confused with inclusion scenes more typical of Russian emerald. Internal growth features are typically straight with angular color zoning following crystal prism faces. Some stones exhibit roiled growth (figure 42) that is distinct from the gota de aceite growth seen in Colombian emeralds. Other mineral inclusions such as brown mica platelets, clinochlore, magnetite spinel, calcite, quartz, and talc can be observed in Ethiopian emerald.
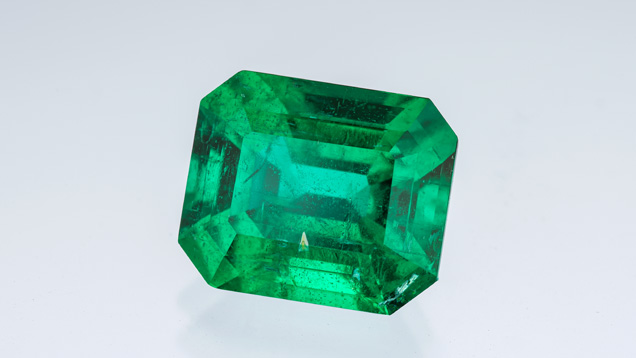
The Internal World of Russian Emeralds. Fine emeralds have been produced from the Ural Mountains since the mid-nineteenth century, placing Russia as one of the classic sources. Russian emeralds can harbor unique inclusion scenes. Iridescent thin films that lie parallel to the basal pinacoid are especially indicative of origin (figure 43). Fluid inclusions take on different forms including elongate (figure 44), irregularly edged multiphase (figures 45 and 46), to blocky (figure 47), although blocky fluid inclusions are uncommon in Russian emeralds. Some fluid inclusions also have patchy, granular fringes (figure 48). Long needles or growth tube inclusions can also be found (figure 49). Brown mica (figure 50) and rod-shaped or needle-like amphibole crystals have been observed. Russian emerald typically has few inclusions, and origin determination can be challenging.
Inclusion Scenes Gone Wrong. Owing to their similar geological genesis, emeralds from Zambia (Kafubu), Brazil, Russia, and Ethiopia may be difficult to distinguish. Making an accurate origin determination requires an experienced gemologist with a robust reference database to compare against unknown stones. Reaching an origin conclusion is easier when the stone has abundant inclusions as opposed to a clean stone. However, every emerald must be observed carefully, as stones from geographically disparate deposits can have similar inclusion scenes. For example, how would you determine origin on these blocky inclusions shown in figures 51 and 52? One is from Zambia and the other from Ethiopia. Irregular fluid inclusions that might have once given an impression of Zambian origin (again, see figure 24) are now also occasionally found in Ethiopian emerald (figure 53). Elongate or thin rod-like fluid inclusions were first seen in Brazilian stones, but similar inclusions have been found in emeralds from Ethiopia and Russia (figure 54). Similarly, rain-like inclusions were once considered diagnostic for Brazilian emerald but can now be observed in Ethiopian and Russian emeralds as well (figure 55). Clusters of brownish mica are common inclusions in all of these schist-hosted emeralds (figure 56). In this class there are no mineral inclusions that can be used to indicate origin. In many cases it is impossible to give an origin opinion based on microscopy. For emeralds from schist-host rock, UV-Vis-NIR analysis is not useful in separation as they all display similar spectra. In this case, chemical analysis by LA-ICP-MS is required to make an accurate origin determination.
Trace Element Chemistry. Trace element analysis is a much more powerful tool for origin determination of emeralds than it is for gem corundum. This likely has its roots in the respective crystalline structures. Beryl has several unique crystal sites of varying sizes and geometry into which trace elements can substitute. This allows easier incorporation and greater variety of substitutional trace elements than for corundum, which has only one crystallographic site that can accept foreign elements. Therefore, emeralds appear to be more sensitive to slight changes in their geological environment, which can impart unique trace element signatures for stones from different geographic localities. Given the overlapping inclusion scenes for emeralds from the deposits discussed here, trace element chemistry analysis is crucial for making accurate origin calls. Several examples of trace element plots used in the GIA laboratory for emerald origin determination are shown in figure 57, and table 1gives the general ranges and averages of the trace elements used in origin determination at GIA. Commonly used trace elements include Li, K, V, Cr, Fe, Rb, and Cs. Colombian emeralds tend to be the purest chemically, possessing generally lower concentrations of the alkalis Li, K, Rb, and Cs as well as Fe. The other hydrothermal/metamorphic emeralds from Afghanistan and China are more enriched in these trace elements, allowing them to be clearly separated from Colombian stones. The most characteristic feature of the schist-hosted emeralds is their enrichment in Fe relative to the hydrothermal/metamorphic group. However, most schist-hosted emeralds also have much higher concentrations of the alkali metals, and members of this group can be generally differentiated from each other by their distinctive trace element profiles. For instance, Russian emeralds tend to have lower Fe and higher Li; Zambian emeralds from Kafubu tend to have high Cs, Fe, and Li; Madagascar emeralds tend to have high K and Fe; Ethiopian emeralds tend to be moderately enriched in most trace elements; and Brazilian stones tend to occupy the lower range of many of the trace elements.
Despite these general trace element profiles, there is still significant overlap when considering only one or two trace elements at a time. However, the selective plotting method employed by GIA for blue sapphires and rubies is also routinely used to provide greater confidence in emerald origin determination based on trace element chemistry; see Palke et al. (2019b), pp. 536–579 of this issue, for a discussion of this method. Essentially, selective plotting makes for an easier comparison between an unknown stone and the vast accumulation of reference data available by filtering out data with dissimilar trace element profiles and comparing only against reference stones that match the full trace element signature of the unknown stone. Elements used in the selective plotting method include Li, K, Fe, Rb, and Cs. The use of selective plotting often allows trace element data to be interpreted more easily and provides greater confidence in origin determination based on these data (figure 58). In most cases trace element chemistry provides solid evidence about a stone’s origin, but sometimes there is still too much overlap in the trace element data (figure 59). When trace element data and inclusion information are ambiguous or contradictory, an “inconclusive” origin determination is always warranted.
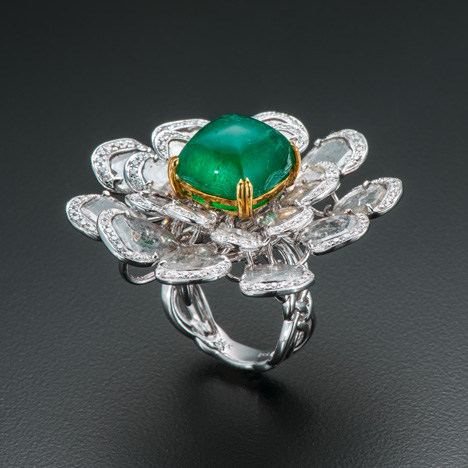
CONCLUSIONS
With the proliferation of sources across the world in the twenty-first century, emerald origin determination has become an increasingly important service offered by gemological laboratories. At GIA, origin determinations are supported by years of meticulous efforts by the field gemology department in assembling a robust reference collection and collecting data on these samples (Vertriest et al., 2019, pp. 490–511 of this issue). This continual accumulation of information has forced the laboratory to adapt its methods and criteria for origin determination to keep up with the evolution of the world emerald market (figures 60 and 61). Given the finding of jagged three-phase fluid inclusions in low-Fe emeralds from Afghanistan and China, any stone that gives an initial impression of a Colombian origin must be carefully scrutinized to avoid inaccurate origin conclusion. The rise of Ethiopian emerald in the last few years has also forced the lab to come to terms with these new stones and their inclusion scenes, which can overlap with Brazilian, Russian, and Zambian emeralds. The lab has also had to understand the increased likelihood of seeing Russian emeralds from the Mariinsky (Malysheva) mine near Ekaterinburg in the Russian Urals. For any non-Colombian emeralds, trace element chemistry is always needed to support an origin determination, given the potential for overlap in their internal features. And yet, despite the years of experience collecting and analyzing data and documenting changes to the emerald mining scene, there are still some stones for which the lab is obligated to issue “inconclusive” origin opinions. Most of the time this is due to a trace element profile that does not match any of the reference data or because of ambiguous or contradictory inclusion scenes. Efforts by the laboratory and the field gemology department will be crucial to closing gaps in our knowledge and keeping on top of further developments in the world of emeralds—whatever the future may hold.
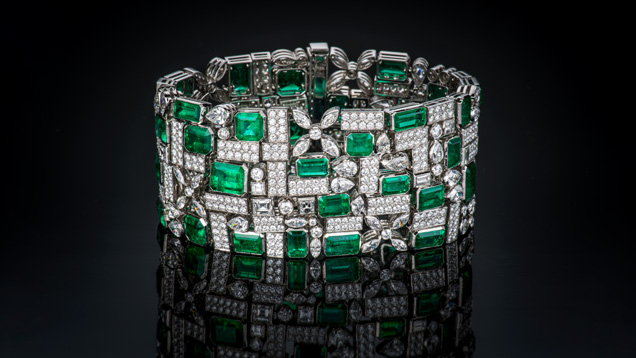