Diamonds from the Deep: How Do Diamonds Form in the Deep Earth?
Carbon is one of the most important elements on our planet, which led the Geological Society of London to name 2019 the Year of Carbon. Diamonds are a main host for carbon in the deep earth and also have a deeper origin than all other gemstones. Whereas ruby, sapphire, and emerald form in the earth’s crust, diamonds form many hundreds of kilometers deep in the earth’s mantle. Colored gemstones tell scientists about the crust; gem diamonds tell scientists about the mantle. This makes diamonds unique among gemstones: Not only do they have great beauty, but they can also help scientists understand carbon processes deep in the earth. Indeed, diamonds are some of the only direct samples we have of the earth’s mantle.
But how do diamonds grow in the mantle? While Hollywood’s depiction of Superman squeezing coal captured the public’s imagination, in reality this does not work. Coal is a crustal compound and is not found at mantle pressures. Also, we now know that diamond does not prefer to form through direct conversion of solid carbon, even though the pressure and temperature conditions under which diamond forms have traditionally been studied experimentally as the reaction of graphite to diamond.
Generally, two conditions are needed for diamond formation: Carbon must be present in a mantle fluid or melt in sufficient quantity, and the melt or fluid must become reduced enough so that oxygen does not combine with carbon (see below). But do diamonds all grow by the same mechanism? What does their origin reveal about their growth medium and their mantle host rock? Surprisingly, diamonds do not all form in the same way, but rather they form in various environments and through varying mechanisms. Through decades of study, we now understand that diamonds such as the rare blue Hope, the large colorless Cullinan, and the more common yellow “cape” diamonds all have very different origins within the deep earth.
Diamonds Form from Fluids in the Mantle That Migrate Due to Plate Tectonics
Diamond is a metasomatic mineral that forms during migration of carbon-bearing fluids, which means that it forms from fluids and melts that move through the mantle. Diamonds can form in both peridotite and eclogite (box A) in the cratonic lithospheric mantle, as well as their higher-pressure equivalents in the much deeper transition zone and lower mantle (box B). Regardless of a diamond’s formation depth, many diamond fluids and melts appear to be related to the recycling of surficial material into the deep earth or to deep melting processes when tectonic plates split apart, or rift, to form new oceans. Both processes occur as part of the geologic cycles that accompany plate tectonics or, in ancient times, some type of pre-plate tectonics.
How Do Lithospheric Diamonds Form?
Lithospheric diamonds (box B) often contain detectable nitrogen, implying that they crystallize from carbon- and nitrogen-bearing (C-N-bearing) fluids. Through the study of diamonds from many different localities, we now know that there are subtle differences in the compositions of these C-N-bearing fluids and melts. These differences manifest as changes in the type of carbon and nitrogen compounds contained in these fluids. “Oxidized” hydrous fluids and melts can contain CO3, CO2, and N2, whereas more “reduced” hydrous fluids contain CH4, NH3, and minor H2.
BOX B: WHERE DO DIAMONDS FORM IN THE EARTH? |
Natural diamonds typically form 150–200 km below the surface of the earth. Diamond formation does not occur everywhere at these depths, but only below the oldest continents that have been stable for billions of years; these areas are known as cratons (see figure 2 in Summer 2018 Diamonds from the Deep). This is because these old cratons all have thick continental roots with cool temperature profiles conducive to diamond formation that geologists term “continental lithospheric mantle.” Diamonds that form within these continental roots are known as lithospheric diamonds and are carried up to the surface of the earth by rare volcanic eruptions known as kimberlites. Other diamonds form much deeper in the earth, in sublithospheric regions of the mantle, below these continental roots. So-called superdeep diamonds form at depths much greater than 200 km, in areas of the mantle known as the transition zone (410 to 660 km below the earth’s surface) and lower mantle (>660 km). After formation they are transported to shallower depths in the mantle, likely through mantle convection cells, and then also brought to the surface by kimberlite eruptions. Earth’s mantle convection associated with plate tectonics is responsible for both the availability of fluids necessary to form diamonds and the return of diamonds to the surface. Ocean floor is thrust deep into the mantle by the process known as subduction and carries diamond-forming fluids in its seawater-altered minerals and rocks. Mantle convection return flow also causes hot mantle to rise to shallower levels and melt, generating the kimberlites that deliver diamonds to the surface. |
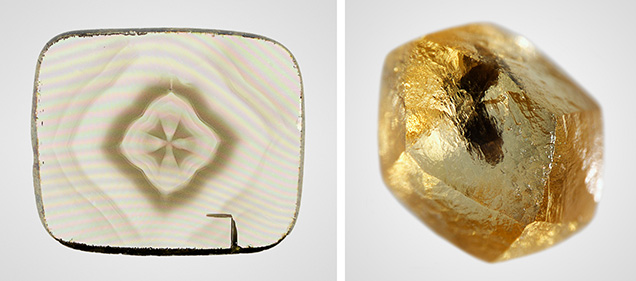
Traditional models for diamond formation from fluids in the mantle invoke either carbonate (CO3) reduction or methane (CH4) oxidation to remove the elements bonded to carbon. Both these mechanisms require some oxygen exchange with the surrounding rocks—peridotite or eclogite (box A)—at the site of diamond precipitation so that elemental carbon can be produced to crystallize diamond. Sometimes peridotite has a limited capacity to exchange oxygen, and we now know that cooling of hydrous fluids containing methane (CH4) and carbon dioxide (CO2) is an alternative way to precipitate diamonds in these rocks (figure 1; Luth and Stachel, 2014; Smit et al., 2016; Stachel et al., 2017).
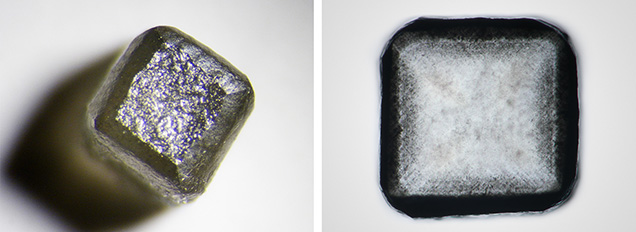
Unfortunately, gem-quality diamonds almost never contain any trapped source fluids that can directly indicate how they formed. However, there are so-called fibrous diamonds (figure 2) that grow much more rapidly than gem-quality diamonds, and even coat earlier gem diamond cores, allowing them to trap abundant fluids. Fibrous diamonds from all over the world, including Canada, West Africa, Siberia, and South Africa, have compositions that are different mixtures of three end-member fluid compositions: carbonate-like, silica-rich, or salty (e.g., Navon et al., 1988; Izraeli et al., 2001, 2004; Schrauder and Navon, 1994; Smith et al., 2012; Weiss et al., 2013; Timmerman et al., 2018).
The origins of fibrous diamonds and their fluids are still debated. Some researchers argue that fibrous diamonds may be related to the kimberlite magmas that transport diamonds to the surface. If so, fibrous diamonds could form right before the kimberlite eruption to the earth’s surface, which would make them much younger than many gem-quality diamonds that may have resided in the mantle for millions to billions of years. One study, however, linked the full range of fluids in some fibrous diamonds from northern Canada to subducting oceanic lithosphere, concluding that the original subducting fluid was related to >200-million-year-old recycled sea water (Weiss et al., 2015). This implies that some fibrous diamonds could have longer mantle residence times and are not necessarily always related to kimberlite eruption.
Fluids similar to those in fibrous diamonds have been found along twin planes in rare gem-quality diamonds from South Africa, indicating that some gem-quality diamonds may have similar formation conditions to fibrous diamonds (Jablon and Navon, 2016). However, fluid inclusions in gem-quality diamonds are actually very rare, and source compositions and the nature of reactants to make diamond must be inferred from the covariation of carbon isotope composition and nitrogen content, either in individual diamonds or diamond suites (Deines, 1980; Stachel et al., 2009). These carbon isotope studies suggest that gem-quality diamonds may form through varying mechanisms, though they typically involve hydrous carbon-bearing fluids that also contain oxygen and hydrogen (either as CO3, CO2, or CH4).
Carbon isotope studies can infer whether a diamond source fluid is “oxidized” or “reduced” (see above) but do not reveal the fluid’s full elemental composition. Diamonds are comprised mostly of carbon, and any other elements present in the diamond are normally at incredibly low abundances that are difficult to measure. For this reason, trace-element data for gem-quality diamonds are only now being realized with improvements in analytical sensitivity. But the available trace-element data for gem-quality diamonds do appear to show many compositional similarities to fluid-rich fibrous diamonds (Krebs et al., 2019), further suggesting that fibrous and gem-quality diamonds crystallize from similar types of mantle fluids.
Diamonds: The Product of Very Deep Subduction
So-called superdeep diamonds that form in the sublithospheric mantle (box B) contain very little nitrogen and are relatively pure, making them nearly colorless (e.g., CLIPPIR diamonds such as the Cullinan diamond and the Constellation diamond from Karowe mine in Botswana; Smith et al., 2016). Some superdeep diamonds are plastically deformed, making them pink or brown (e.g., diamonds from the Juína area in Brazil). Rarely, they can contain some boron imparting a blue color (e.g., the Hope diamond; Smith et al., 2018). Based on studies of inclusion-bearing superdeep diamonds, we now know that they cannot all form in the same environment and that they must crystallize from a wide variety of source fluids and melts that all relate to different aspects of very deep subduction (box B).
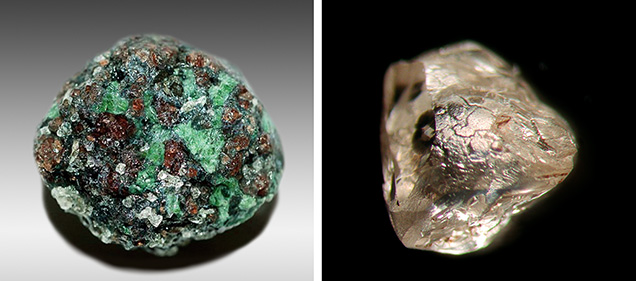
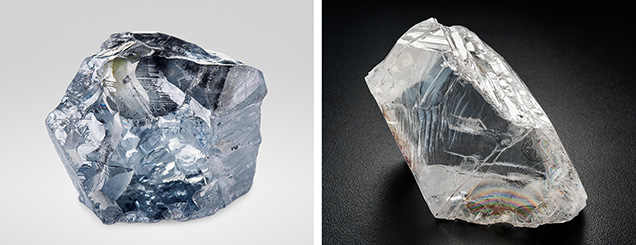
Many superdeep diamonds, such as those from the Juína and Machado River areas in Brazil, preserve inclusion evidence for their crystallization from carbonate-rich fluids (figure 3; Walter et al., 2008; Bulanova et al., 2010; Burnham et al., 2016; Thomson et al., 2016). These CO3-rich source fluids likely derive from oceanic lithosphere that was deeply subducted into the mantle transition zone (box B). CLIPPIR diamonds (figure 4) are now also known to crystallize from metallic melts in the deep earth that are associated instead with reduced carbon (CH4) and hydrogen (Smith et al., 2016). These diamonds have carbon isotopic compositions that are unlike those expected for the mantle, but rather indicate an ultimate source for the carbon in the oceanic lithosphere, as do other superdeep diamonds. The third kind of superdeep diamond, boron-containing blue diamonds, are also related to deeply subducted oceanic lithosphere (figure 4; Smith et al., 2018). In this case, however, the source for the boron-containing fluids is likely to be altered peridotite in the deeper portions of the subducted lithosphere.
Our Fluid Understanding of How Diamonds Form: Future Studies of Diamond-Forming Fluids
Our understanding of the fluids from which diamonds form is improving with advances in the sensitivity of analytical techniques. Most diamonds are only comprised of carbon, with less than a few parts per million of nitrogen, boron, and other trace elements. Many elements that scientists are interested in detecting in diamond are currently not able to be measured accurately. With improved analytical techniques that can measure the very low concentrations of trace elements in diamonds, we may be able to better understand the different origins for diamond source fluids.
Future work will also reveal how the different diamond-forming environments relate to each other. Our understanding of the origin of lithospheric diamond source fluids is better developed than it is for sublithospheric diamonds. This is because they contain much more nitrogen that can be studied along with carbon, and sometimes they are found still trapped within their peridotite or eclogite host rocks (figure 3). Since superdeep diamonds contain little or no nitrogen, and are never found within their original superdeep mantle host rocks, they provide less information about the geological conditions by which they formed. We do know, however, that the carbonate-rich fluids, metallic melts, and boron-rich fluids in superdeep diamonds all relate in some way to deeply subducted material from the earth’s oceanic lithosphere. But the challenge remains for geologists to predict where these domains, all with different oxidation states, occur in the deep earth. Current diamond exploration models have a near-exclusive focus on lithospheric diamonds, so geologists still need to do some work to figure out how to explore for potentially high-value superdeep diamonds.