Inclusions in Gemstones

Gem inclusions are mineral crystals or cavities filled with fluid and/or gas that occur in a host gemstone. Many gems contain microscopic inclusions (ranging in size from >1 mm down to submicroscopic nanoscale inclusions) that can reveal much about the host material. As a result, the use of the microscope (or loupe) to examine these inclusions offers one of the most important methods available for gem identification.
Inclusions are important for determining the natural, synthetic, or treated character of a gem, and for establishing the likely geographic origin of a valuable colored stone. In addition to their usefulness for identification, inclusions cause certain distinctive and desirable optical phenomena such as asterism and chatoyancy, as well as some of the features used in clarity grading. In this new installment of “Colored Stones Unearthed,” we will discuss inclusions in gems—how they form, how they are studied, and what they mean not only for gemologists but also for geoscientists.
BRIEF HISTORY OF INCLUSION STUDIES
While the study of inclusions might seem to be a modern concept requiring the use of advanced microscopes with sophisticated optical lenses, early naturalists did have some basic knowledge of how inclusions could be used to understand the geological history of a gem. Consider amber, for instance. By observing insects trapped in amber, Pliny the Elder was able to conclude that amber represents fossilized tree resin (Ball, 1950) (figure 1).
However, it was only much later that inclusions began to be classified and studied in a more systematic fashion. The pioneering scientist Robert Boyle was perhaps one of the first to describe inclusions in gems—“a specimen of quartz with a cavity containing a fluid with a moveable gas bubble, and reddish brown hair-like inclusions in amethyst” (Boyle, 1672). With the improvements in design and functionality of optical microscopes beginning in the late 1700s, scientists started to use them to examine tiny features in rocks and minerals (Kile, 2003, 2013). Déodat de Dolomieu discussed the presence of a hydrocarbon fluid (petroleum oil) in quartz in 1792. In 1819, Chester Dewey detailed a quartz specimen from Massachusetts that displayed a cavity containing a liquid with a moveable bubble and several black or brown particles (1818, pp. 345–346). Noted scientists Humphry Davy (1822), David Brewster (1826, 1827, 1844, 1845a,b, 1863), and William Nicol (1828) each described minerals that contained inclusions consisting of one or more fluids and moveable gas bubbles. In 1854, Johann Reinhard Blum and his coauthors published a book discussing various mineral inclusions they had observed. By linking observations on mineral inclusions and host rock formation, Henry Clifton Sorby (1858) became one of the founders of the geological science of petrography with an article titled “On the microscopical structure of crystals, indicating the origin of minerals and rocks.” An 1862 article by Gustav Rose identified the presence of oriented, needle-like inclusions, such as those seen in the star ruby in figure 2, as the cause of asterism in minerals. The first descriptions of inclusions specifically in gem minerals were published in several articles by Henry Sorby in 1869 and by Isaac Lea between 1866 and 1876. Other reports on solid and fluid inclusions in various minerals followed (e.g., Hartley, 1876, 1877).
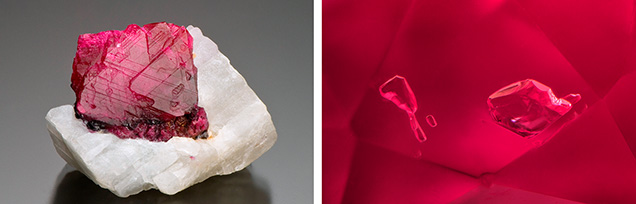
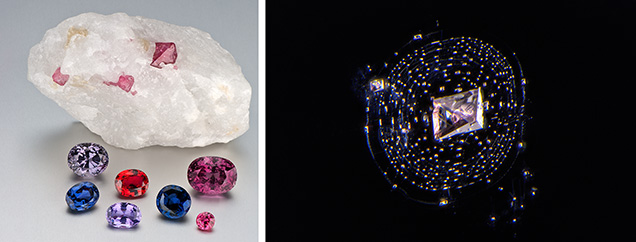
The use of inclusions to understand a gem’s geological growth environment can be understood with the examples shown in figures 3–6. Ruby derived from extremely pure calcite (CaCO3) marble, as in the deposits of Mogok, Myanmar, often contains calcite inclusions (figure 3), whereas magnesium-rich spinel from the same geological region in Mogok will likely contain magnesium-rich dolomite [CaMg(CO3)2] (figure 4). Similarly, emeralds from mica-rich rocks called schists, such as Russian emeralds, often contain mica inclusions (figure 5). On the other hand, emeralds from the hydrothermal deposits in Colombia, where the gems occur in veins rich in carbonate, quartz, and pyrite, often contain inclusions of carbonate minerals. Figure 6 shows an exceptional example of a carbonate inclusion, which itself contains a pyrite inclusion that perfectly matches the mineralogy seen in many emerald-bearing hand samples from Colombia.
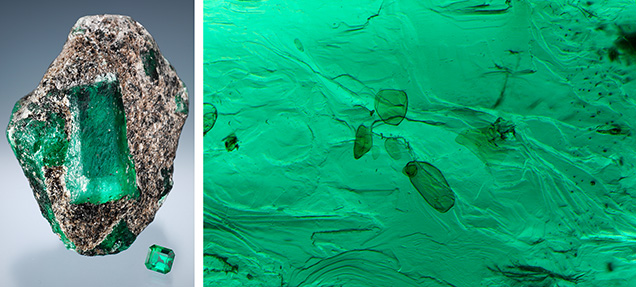
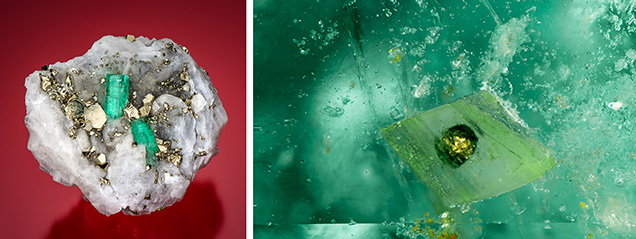
Beginning in the 1940s, the well-known European gemologist Eduard Gübelin began publishing a series of important articles in Gems & Gemology and Journal of Gemmology on inclusions and the evidence they could provide on the geologic and geographic origin of the host gemstone (e.g., Gübelin, 1944–1946, 1948, 1950, 1953, 1969, 1974). Dr. Gübelin’s publications were followed by several important books on gem inclusions coauthored by John Koivula (1986, 2005, 2008) that have been widely used and appreciated by the gemological community. Hollister and Crawford (1981), Roedder (1984), Samson et al. (2003), and Chi et al. (2020) contributed technical summaries on fluid inclusion research.
INCLUSIONS IN MINERALS AND GEMS
Minerals frequently contain small inclusions of foreign materials (solids, liquids, and gases) that were trapped during mineral formation, and these can be seen with magnification. Crystals that formed during metamorphism by solid-state recrystallization, or almost entirely in the solid state without significant involvement of fluids, can display solid inclusions. Those that occur in igneous and sedimentary rocks were formed in the presence of a geological fluid and, as a result, can contain single or multiphase inclusions of that fluid (as well as solid or vapor inclusions). These geological fluids consist of high-density silicate or carbonatite melts, low-density water-rich fluids or vapors, and, in rare cases, organic hydrocarbons (oils). Following crystallization, the mineral crystals in all of these rock types can become cleaved or fractured one or more times, and these cleavages and fractures can later become healed in the presence of liquid or gaseous fluids. Tiny amounts of these fluids can remain as inclusions along the healed zone (figure 7). The crystal form of the host mineral typically determines the morphology of these fluid inclusions. Fluid inclusions usually have a geometric, angular shape that reflects the typical crystal forms of the host. For this reason, these fluid inclusions are often referred to as “negative crystals.”
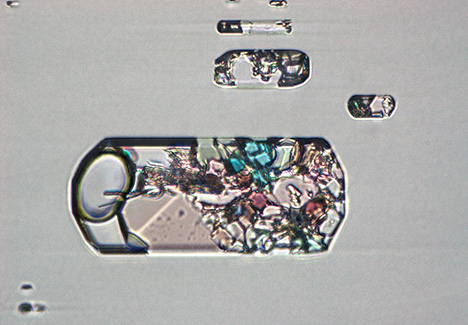

While either solid or liquid at the time of their original entrapment in crystals at high temperatures in the earth, inclusions can undergo phase changes during cooling of the host crystals as the host rocks are brought toward the earth’s surface. Near the surface, inclusions of fluids normally remain as fluids, while melt inclusions normally solidify to some type of glass or other solid (figure 8). In some cases, a fluid can separate into two fluids that are immiscible (i.e., do not mix with one another at cooler temperatures). Solid inclusions can also undergo changes to lower-density or secondary alteration phases.
At elevated temperatures in the earth, minerals can accommodate greater amounts of foreign impurity elements in their crystal structures. But when the minerals cool in the earth’s crust, these impurity elements can no longer be contained, and they are usually expelled from the structure (exsolved) as inclusions of different minerals (such as rutile needles in sapphire). At lower pressures and temperatures near the earth’s surface, gases originally dissolved in inclusion fluids can also be exsolved as distinct gas bubbles within water or carbon dioxide or some other fluid. Secondary or “daughter” crystals can also form by coming out of the solution from the fluid in the inclusion (figure 7).
Inclusions occur either individually or in small groups. Sometimes they are abundant enough to affect the transparency of the host mineral. They often occur randomly in the host crystal. But they may also form along certain crystallographic directions, color zones, or healed fractures, or they can occur in geometric patterns related to the crystal symmetry of the host. Solid inclusions can exhibit their own crystal shape, have a rounded or irregular appearance, or adopt the negative crystal shape of the host mineral. Gemologists categorize inclusions based on their appearance (shape, size, transparency, color, luster, contrast with the host, and orientation) and their association with other mineral inclusions.
Inclusions and their host gemstones can have various age relationships with one another, although this formation relationship cannot always be clearly established based on visual or other evidence. Protogenetic primary solid inclusions were present before the host mineral formed, and the mineral grew around and entrapped them—these may display irregular or partly dissolved shapes. Syngenetic primary solid, liquid, or gas inclusions formed at the same time as the host mineral by being trapped on growing crystal faces. Solid inclusions sometimes have well-formed crystal shapes that represent either their morphology or a morphology imposed on them by the host. Epigenetic secondary inclusions formed after the host by exsolution along a rehealing cleavage or fracture.
SCIENTIFIC STUDY AND GEOLOGICAL IMPORTANCE OF INCLUSIONS
The study of solid and fluid inclusions provides a way for the scientist to reconstruct events and processes in the geological past. Fluid inclusions represent actual, and often quite rare, samples of the geological fluids that existed at some time in the history of rocks and minerals. As such, they can provide information on the physical and chemical conditions that were present during and after rock formation. In particular, studies of inclusions can reveal information on:
Temperature: When a mineral and the fluid inclusions within it cool over geologic time, they shrink at different rates. The inclusion fluid shrinks faster than the solid crystal host, and this difference is evident in the exsolution over time of a gas bubble within the fluid at temperatures existing near the earth’s surface (see figure 9). Through gradual, controlled heating of the crystal sample and observing when the gas bubble disappears back into the fluid (homogenizes), one can estimate the temperature that existed when the inclusion itself first formed.
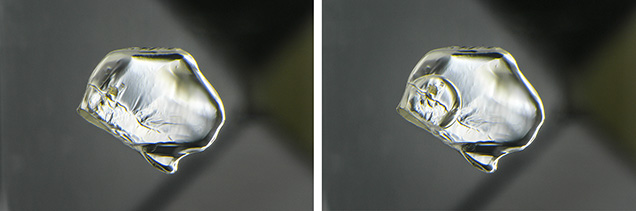
Pressure: Once the chemical composition of the fluid inclusions is identified, scientists can use experimental laboratory data on similar fluids to get a sense of the pressures that existed at the time and the environment in which the inclusions were trapped in the host mineral.
Density: With data on chemical composition, along with the density and individual volume of the various phases present in a fluid inclusion, the total average density of all the phases in the inclusion can be calculated. This result is important for understanding the types of fluids and their circulation in the earth’s crust.
Chemical Composition: The liquids, the solids, and in some cases the gases present in inclusions can be analyzed to obtain information on the chemical environment of inclusion formation in the earth’s crust and mantle. Inclusions provide important geological information on the deep earth that may not be available from any other source.
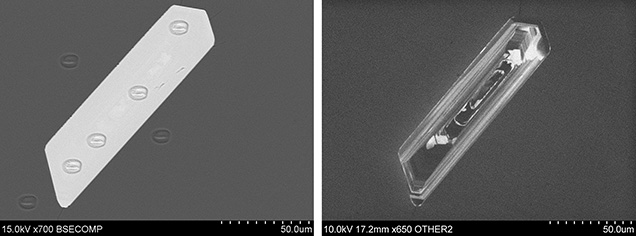
Geologic Age Dating: When solid inclusions in mineral crystals contain small amounts of radioactive trace elements, it is possible to determine the geologic age of the inclusion (figure 10). Radioactive elements undergo a specific decay over known periods of geologic time, so careful determination of these elements can be used to calculate mineral formation ages.
Application of Inclusion Research: The abundance and frequent occurrence of inclusions in a variety of geologic samples offers numerous opportunities for inclusion studies that support a multitude of geological investigations, such as:
- Verifying the geologic conditions of formation of ore deposits, providing a tool for the field exploration of new deposits
- Determining the conditions of formation, mineralogy, and geologic history of rocks found in many types of crustal and upper mantle environments (some results cannot be obtained any other way)
- Inspecting fluid inclusions that contain hydrocarbons, which can be important for oil exploration
- Determining the metamorphic or magmatic provenance of certain minerals found today in sediments and sedimentary rock formations
- Ascertaining the conditions and geological ages of diamonds and coexisting mineral phases in the lower crust and mantle
- Hypothesizing the conditions of geotectonic events such as crustal formation and subduction, mountain building and erosion, volcanism, metamorphism, and sedimentation
- Studying trapped organisms and vegetation found as fossil inclusions in amber to provide information on the ancient biosphere
- Using gases trapped in inclusion fluids to study the ancient atmosphere
GEMOLOGICAL IMPORTANCE OF INCLUSIONS
For the gemologist, inclusion studies combined with documentation of standard physical properties provide the basis for gem identification. In some instances, particular inclusions uniquely identify a gemstone and its geographic origin. Observation of inclusions is carried out using a gemological microscope and various lighting configurations. These may include brightfield, darkfield, fiber-optic lighting at different orientations to the sample, shadowing, use of polarizing or colored filters, and other image-processing techniques. Since the 1950s, inclusion photography has been an essential part of gemological education (see Koivula, 1981, 2003; Renfro, 2015a,b).
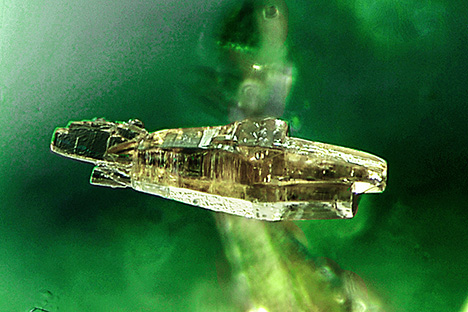
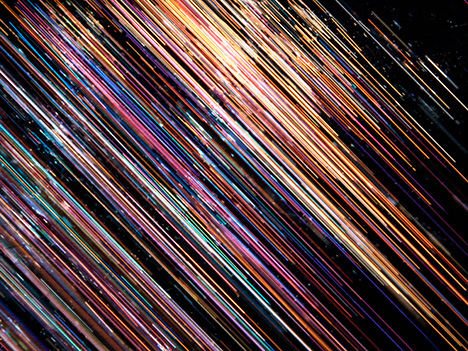
Inclusions are often the key to determining the geographic origin of gemstones. Some gems contain inclusions that are very specific to certain localities. Parisite inclusions in an emerald are a diagnostic indicator of Colombian origin (figure 11). In other cases, inclusions are one piece of the puzzle, such as the long, slender rutile silk seen in a Sri Lankan sapphire (figure 12).
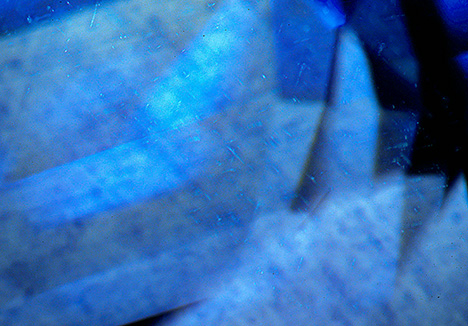
Inclusions are an important clue in identifying treatments applied to gemstones. The observation of internal diffusion of blue color around relict rutile silk in a sapphire offers evidence of high-temperature heat treatment meant to enhance the stone’s blue color (figure 13). Microscopy is arguably the most important way to identify many treatments such as heat treatment, dyes, and clarity enhancement through fracture filling.
INCLUSIONS AS NATURAL ART
Inclusions in gems serve as visual works of natural art (figure 14). Fortuitous geological processes in the earth created these inclusions in natural, untreated gems without any human intervention. Their shape, appearance, color(s), and in some cases the constraints imposed by the crystal symmetry of the mineral host all combine to create an inclusion “scene” that is entirely unique and never to be repeated. The artistic nature of inclusions is perhaps best illustrated by a series of photomicrograph charts created by Nathan Renfro and others that have appeared in Gems & Gemology since 2016 (Renfro et al., 2016; 2017a,b; 2018; 2019; 2021).

The field of gemological research exists at a fascinating intersection between the cold, dispassionate scientific method and the impassioned and provocative world of aesthetics and art. So perhaps it is fitting that these inclusions that tell us so much about the history and genesis of gems can also move and touch us as works of art. Future installments will further explore the ways in which scientific inquiry of the geology of colored stones can deepen our appreciation of these gems.