Black Nephrite Jade from Guangxi, Southern China
ABSTRACT
Twelve black nephrite samples collected from the Guangxi region of southern China were investigated by standard gemological testing, polarized microscopy, scanning electron microscopy, and electron microprobe analysis, as well as infrared, Raman, and ultraviolet/visible/near-infrared spectroscopy. Originating from Ca-skarn contact metasomatism between limestone bearing siliceous rock and diabase intrusions, black nephrite from Guangxi consists mainly of actinolite or ferro-actinolite and minor stilpnomelane, andradite, apatite, epidote, quartz, diopside, pyrrhotite, and pyrite. Its high refractive index (1.625–1.650) and specific gravity (3.015–3.405), as well as its black color in natural light for plate samples and greenish yellow/brownish yellow or pale green/yellowish green/green color in transmitted light for thin-section samples, are primarily attributable to a high iron content: 11.67–25.75 wt.% Fe oxides and a Mg/(Mg + Fe2+) ratio of 0.765–0.343. Mid- and near-infrared spectra and Raman spectra are characterized by four vibration bands corresponding to hydroxyl groups coordinated to three cations in M1 and M3 positions for (M1M1M3)OH combinations (Mg)3OH, (Mg2Fe2+)OH, (MgFe2+2)OH, and (Fe2+)3OH. Their relative intensities further indicate different iron contents and isomorphous substitution of Fe2+ for Mg2+ ions.
INTRODUCTION
Nephrite is an essentially monomineralic rock composed primarily of tremolite-actinolite [Ca2(Mg,Fe)5Si8O22(OH)2] amphiboles in a felted microcrystalline habit (Leake et al., 1997), making it greasy, tough, and easy to carve. Nephrite mainly forms in two settings (Harlow and Sorenson, 2005; Harlow et al., 2014). The first is dolomite replacement by silicic fluids commonly associated with granitic plutonism (i.e., D-type). Important deposits occur at Xinjiang, China (Liu et al., 2011a, 2015); Chuncheon, South Korea (Yui and Kwon, 2002); and Cowell, South Australia (Flint and Dubowski, 1990). The other type of setting is serpentinite replacement by Ca-metasomatism at contacts with more silicic rock (i.e., S-type). Related deposits include Siberia, Russia (Prokhor, 1991); South Island, New Zealand (Wilkins et al., 2003); British Columbia, Canada (Leaming,1998); and Fengtien, Taiwan (Yui et al., 2014). Nephrite found in the southern Chinese region of Guizhou forms in a different setting, within the metasomatic zone between diabase and limestone bearing siliceous rock (Yang, 2013).
In 2011, a new nephrite deposit was discovered in southern China at Yantan Town, Dahua County, in the Guangxi Zhuang Autonomous Region (Li et al., 2011). The Guangxi deposit is separated from the Guizhou deposit by the Hongshui River. Nephrite from Guangxi comes in a wide variety of colors such as white, green, brown, and black, and some varieties exhibit either a banded structure or a dendritic pattern (Wang et al., 2012; Yin et al., 2014). Among them, the nephrite with a homogeneous, pure black color reminiscent of Chinese calligraphic ink is the most prized by jade carvers and traders (figure 1). Several scholars have studied the mineralogy, spectroscopy, and genesis of black nephrite from Guangxi (Wang et al., 2014; Mo and Mao, 2016; Peng et al., 2017). The results suggest that it consists mainly of actinolite or ferro-actinolite and features a high iron content. Wang et al. (2014) proposed that the Guangxi deposit is a skarn-type occurrence and conducted preliminary analysis of its formation process. But few studies have discussed how high iron content influences its gemological properties or vibrational spectra. Moreover, detailed data and systematic research on its formation mechanism are still limited.
This study employed standard gemological testing, polarized microscopy, scanning electron microscopy, electron microprobe analysis, infrared spectroscopy, Raman spectroscopy, and ultraviolet/visible/near-infrared spectroscopy to investigate the gemological properties, petrographic features, microstructures, chemical compositions, and vibrational spectra of black nephrite from Guangxi. The results demonstrate relationships between iron content and gemological properties as well as vibrational spectra. Additionally, the authors propose evidence for its deposit type and discuss the source rocks involved in its formation. This study will help in the identification of black nephrite from Guangxi and provide a basis for further research on the formation mechanism of nephrite from southern China.
GEOLOGICAL SETTING
Located in the intersection zone of the Tethys Tectonic Domain and the Circum-Pacific Tectonic Region, the Guangxi black nephrite deposit is between the southeast margin of the Yangtze Craton and the South China Caledonian Fold Belt, and adjacent to the Sanjiang Orogenic Belt in the southwest (Bureau of Geology and Mineral Resources of Guangxi Zhuang Autonomous Region, 1985; figure 2). According to the geologic map from the Chinese National Geological Archives (figure 3), the strata exposed here consist mainly of marine carbonate rocks deposited from the early Carboniferous to Middle Triassic periods (359–237 Ma). Basic igneous rock (i.e., diabase) emplaced during the late Permian period (260–252 Ma) within the Upper Carboniferous to Permian (323–252 Ma) limestone bearing banded chert/dolomite and Lower Permian (299–272 Ma) siliceous rock/clay rock/limestone bearing banded chert display an obvious circular structure in anticlines1. These anticlines extend northwest to southeast and are controlled or transformed by several faults extending northwest by north, northeast by north, and northeast by east. Black nephrite is situated in the southwest limb of an anticline and occurs at the contact zone between diabase2 and carbonate rock.
According to local miners, the black nephrite was first discovered under houses in the village of Xiannv, and this was considered a secondary deposit. Mining of the primary deposit started in late 2012 but was prohibited by the local government the following year for conservation reasons. Fieldwork for this study was done in August 2017 and August 2018 at the primary deposit, located 538 meters above sea level at 24°01'38.23"N, 107°27'39.61"E. The deposit contained many scattered diabase fragments due to previous mining activities. A small exposed outcrop of marble (figure 4B) and altered marble (figure 4C) was subjected to metasomatic diopsidization, silicification, albitization, and tremolitization/actinolitization. Residual thin-bedded sedimentary sandstone/shale and orebody bearing black nephrite are distributed from top to bottom (figure 4A). Underlying the altered marble is an alteration zone with an obviously banded structure and spatial inhomogeneity (figures 4C and 4D). Typical metamorphic minerals such as garnet, clinopyroxene, stilpnomelane, and amphibole occur in different bands. A black nephrite vein about 30 cm thick was layered in the lower part (figure 4C). A fracture revealed that the jade is very compact and fine-grained (figure 4E). Diabase is mainly distributed under the vein, and the typical texture of the lath-shaped plagioclase crystals set in a matrix of clinopyroxene could be observed in the fracture (figure 4F).
Because of the subtropical climate, a thin yellowish brown to reddish brown weathering crust similar to iron rust is very common at the surface of the rocks in the primary deposit (figures 5A and 5B). The weathering crust on the black nephrite rocks from the secondary deposit has a yellow color like that of loess mud (figures 5C and 5D). Therefore, black nephrite rough mined from the Guangxi primary and secondary deposits is referred to in the market as “iron rust crust rock” and “loess mud crust rock,” respectively.
MATERIALS AND METHODS
Materials. Twelve pieces of black nephrite rough (GB-01 through GB-12) from Guangxi were investigated for this study. GB-01 through GB-09 were supplied by local miners, and GB-10 through GB-12 were collected from the primary deposit during fieldwork. We cut and polished the rough into plate samples, 1.10–6.13 mm thick and weighing 0.84–38.11 ct (see table 1 photos in natural light), and further polished them into thin sections with various thicknesses to meet the requirements of different measurements.
Analytical Methods. All measurements were collected with equipment at the Laboratory of Gem and Technological Materials of Tongji University, unless otherwise specified. The refractive indices of 12 black nephrite plate samples were measured using a refractometer (by normal reading from flat wafers), and specific gravity was measured hydrostatically with an electronic balance. Ultraviolet fluorescence reactions were examined with a long-wave/short-wave UV lamp. Color, luster, and transparency of the plate samples were observed in natural light, and the color of the thin sections (0.73–0.97 mm thick) was examined in transmitted light.
Petrographic features were studied with a Nanjing Jiangnan Novel Optics BM2100 polarized light microscope. Six thin sections (GB-04, GB-05, GB-06, GB-08, GB-09, and GB-10) with a standard thickness of 0.03 mm were observed in both plane- and cross-polarized light. Microstructures on the fractured surface of sample GB-06 were observed using a Hitachi S-4700 scanning electron microscope (SEM) with an accelerating voltage of 15 kV at the Analysis and Testing Center of Suzhou University.
Backscattered electron (BSE) images and chemical compositions of minerals in 12 black nephrite thin sections (0.03 mm thick) and plate samples were obtained with a JEOL JXA-8230 electron microprobe at the State Key Laboratory of Marine Geology of Tongji University using an accelerating voltage of 15 kV, a beam current of 10 nA, and a beam diameter less than 5 μm in wavelength-dispersive spectrometry mode. Natural and synthetic mineral standards (SPI Supplies) were used to calibrate all quantitative analyses, and a ZAF program was used for data reduction. Two to six points per sample/mineral were analyzed. Cations per formula unit of amphiboles were calculated based on 23 oxygen atoms and 15 cations. The total iron content was first estimated as FeO, and Fe2+/Fe3+ ratios were then estimated by a charge-balance method on a stoichiometric sum of cations (Schumacher and Wang, 2001).
Infrared absorption spectra of 12 plate samples were obtained with a Bruker Tensor 27 Fourier-transform infrared spectrometer. For measurements in the 4000 to 400 cm–1 range, nearly 1.5 mg of powder was scraped from each sample and dispersed in 150 mg of KBr to prepare pressed pellets. In the 8000 to 4000 cm–1 range, thin-section samples (0.73–0.97 mm thick) were tested directly. Both types of spectra were recorded in transmission mode at a resolution of 4 cm–1, a scanning frequency of 10 kHz, and 32 scans. Infrared spectra in the 3700 to 3600 cm–1 range were obtained using a Bruker Lumos micro-Fourier-transform infrared spectrometer in attenuated total reflectance mode with medium pressures, at a resolution of 4 cm–1 and 32 scans per area (150 × 150 μm2).
Raman spectra of 12 plate samples were collected using a Horiba Jobin Yvon LabRAM HR Evolution confocal micro-Raman spectrometer equipped with a 532 nm Nd:YAG laser. The laser was focused on the mineral surface using an optical microscope with a 100× objective, and the laser power was around 50 mW. Depolarized spectra in the 4000 to 100 cm–1 range were acquired using a grating with 600 gr/mm, a scan time of 20 s, and 3 scans.
Ultraviolet/visible/near-infrared (UV-Vis-NIR) spectra of 12 thin sections (0.13–0.49 mm thick) were obtained with a GEM-3000 spectrophotometer at a resolution of 1 nm, an integration time of 80 ms, a smoothness degree of 2, and 30 scans.
RESULTS
Gemological Properties. The gemological properties of the 12 nephrite samples from Guangxi are listed in table 1. All plate samples (1.10–6.13 mm thick) were black and opaque and exhibited a greasy to vitreous luster in natural light. The thin sections (0.73–0.97 mm thick) showed two different kinds of mottled color in transmitted light. Three samples (GB-01, GB-02, and GB-10) were greenish yellow to brownish yellow, while the remaining nine ranged from pale green to yellowish green to green. All samples were inert to both long- and short-wave UV radiation and displayed an RI of 1.625 to 1.650 and an SG of 3.015 to 3.405. In addition, different amounts of minor minerals were included in almost every sample. The most obvious were minerals with a typical metallic luster and brassy yellow color in plate samples GB-08 and GB-09.
Petrographic Features and Microstructures. Polarized optical images (figures 6A and 6B) combined with electron microprobe data (table 2) showed that the black nephrite samples from Guangxi consisted mainly of fine-grained, felted actinolite with first-order orange to second-order bluish green interference colors in cross-polarized light. (For more on orders of interference colors, see box A.) Faint light yellow/light green pleochroism was observed in plane-polarized light. SEM images (figure 6C) further indicated that the actinolite fibers were nearly 20 × 0.5 μm in size. Some fibers formed either a radial (figure 6B) or a parallel (figure 6C, bottom) structure. Polarized optical images and BSE images (figure 7) combined with electron microprobe data (table 3) revealed various amounts of Fe sulfides (up to 30%), stilpnomelane (up to 25%), diopside (up to 15%), quartz (up to 8%), epidote (up to 5%), andradite (up to 5%), and apatite (up to 3%) (table 1).
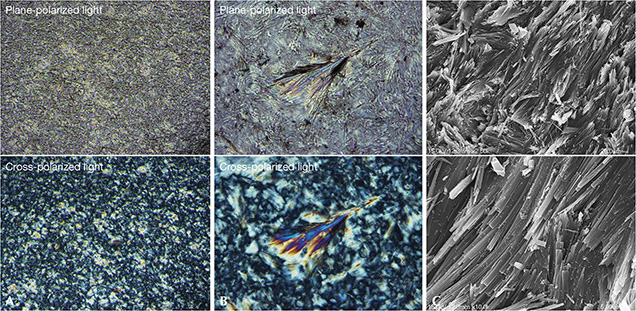
Box A: Orders of Interference Colors |
The order of interference color is used to describe colors observed in thin sections with a petrographic microscope when identifying minerals, particularly anisotropic minerals. There is a path difference caused by the difference in velocity between the two rays resolved by the anisotropic (doubly refractive) substance between crossed polarizers, which is known as the retardation. The retardation (r) increases linearly with both the thickness (t) of a sample and the birefringence (n2–n1): r = t (n2–n1). When the two rays enter the upper polarizer, the retardation results in destructive interference for certain wavelengths to give the interference colors. To distinguish the colors produced by different multiples of wavelengths, the interference colors are divided into “orders,” with the end of each order marked by a red color representing one full wavelength retardation (Delly, 2003). For example, the first order starts with black and gray, followed by yellow and orange, and ends with “first-order red.” The second order is made up of violet, blue, green, yellow, orange, and “second-order red.” Interested readers should refer to the Michel-Lévy chart (see Delly, 2003), which shows the interrelationships between thickness, birefringence, and interference colors. |
Chemical Compositions. Average chemical compositions and cations per formula unit of amphiboles for the 12 samples are shown in table 2. According to Leake et al. (1997) and Hawthorne et al. (2012), amphiboles in black nephrite belong to the calcic group, characterized by 7.898–8.080 atoms per formula unit (apfu) of Si, 0.004–0.156 apfu of K on the A site, and 1.703–1.899 and 0.013–0.047 apfu of Ca and Na on the B site, respectively. In addition, the Mg/(Mg + Fe2+) ratio of amphiboles in GB-07, GB-08, GB-09, and GB-12 ranged from 0.343 to 0.465, and these were identified as ferro-actinolite. The ratio ranged from 0.511 to 0.765 in the remaining samples, which were identified as actinolite. Therefore, the black nephrite from Guangxi was composed mainly of actinolite or ferro-actinolite. All samples contained abundant iron (11.67–25.75 wt.% FeO) and minor manganese (0.16–0.33 wt.% MnO), titanium (below 0.03 wt.% TiO2), and chromium (below 0.02 wt.% Cr2O3).
Representative chemical compositions of minor minerals including andradite, epidote, stilpnomelane, quartz, diopside, apatite, pyrrhotite, and pyrite (figure 7) are shown in table 3. The andradite was composed mainly of CaO (33.14–33.28 wt.%), FeO (26.37–28.09 wt.%), and SiO2 (35.51–37.28 wt.%). The stilpnomelane (brown in GB-05 and green in GB-08) was characterized by a high iron content (30.92 and 34.00 wt.% FeO, respectively). In diopside, iron was lower and magnesium was higher in the core (table 3, GB-04-1) than in the rim (table 3, GB-04-2), which explains its zoning structure of darkness and brightness in the BSE image (figure 7E). The pyrrhotite in sample GB-09 had a typical chemical composition of 61.14 wt.% iron and 39.77 wt.% sulfur, while the pyrite’s was 47.52 wt.% iron and 52.29 wt.% sulfur.
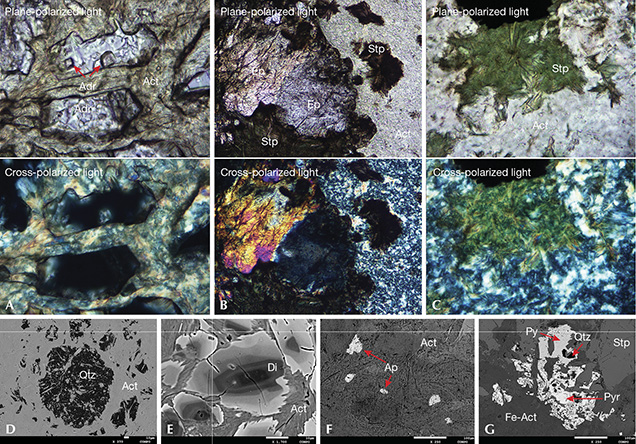
Infrared Spectroscopy. Mid-Infrared Spectroscopy. Mid-infrared spectra (figure 8) of the black nephrite plate samples were characterized by one set of bands in the 3700 to 3600 cm–1 range and three sets (1200–800 cm–1, 800–600 cm–1, and 600–400 cm–1) in the 1600 to 400 cm–1 range, typical of M3-OH (M is an abbreviation for a metal ion) and [Si4O11] vibrations in actinolite or ferro-actinolite, respectively (Wen et al., 1988). The asymmetric and symmetric stretching modes of the Si-O-Si and O-Si-O groups appear mainly at approximately 1096, 1051, 1012, 993, 951, 919, 883, 751, 688, and 645 cm–1. The bands near 504 and 463 cm–1 are related to the bending modes of the Si-O group, stretching modes of the M-O group, and translational modes of the O-H group. The bands around 3681, 3664, 3646, and 3625 cm–1 are related to the asymmetric stretching modes of the M3-OH group. No absorption bands related to minor mineral phases were observed.
Near-Infrared Spectroscopy. Near-infrared spectra (figure 9) of the black nephrite thin sections were mainly characterized by the combination modes of the M3-OH, Si-O groups, or water and the multiple vibration modes of the hydroxyl group in actinolite or ferro-actinolite. Four strong and sharp bands around 7185, 7156, 7117, and 7078 cm–1 are related to the first overtone of M3-OH asymmetric stretching modes (Burns and Strens, 1966). The broad band centered at nearly 5226 cm–1 is assigned to the combination modes of water adsorbed within the actinolite or ferro-actinolite aggregates. The combination modes of the M3-OH and Si-O groups appear at approximately 4295 and 4161 cm–1.
Raman Spectroscopy. Raman spectra (figure 10) of the black nephrite plate samples were primarily characterized by peaks in the 3700 to 3600 cm–1 and 1200 to 100 cm–1 ranges, which are typical of M3-OH and [Si4O11] vibrations in actinolite or ferro-actinolite. Peaks at approximately 1060, 1047, 1024, 946, and 927 cm–1 are related to the stretching modes of the Si-O group, and those at 739 and 665 cm–1 are related to the stretching modes of the Si-O-Si group. The bending modes of the Si-O group appear mainly at approximately 522 and 431 cm–1. Peaks at around 408, 379, 355, 330, 219, 171, 143, 128, and 112 cm–1 are assigned to the lattice vibration modes. Four sharp Raman peaks near 3674, 3661, 3643, and 3624 cm–1 are related to the stretching modes of M3-OH groups (Lu, 2005; Feng et al., 2017).
UV-Vis-NIR Spectroscopy. Black nephrite thin sections (0.13–0.49 mm thick) with greenish yellow to brownish yellow (samples GB-01, GB-02, and GB-10) and pale green to yellowish green to green (samples GB-04, GB-06, and GB-09) colors in transmitted light exhibited similar but slightly different absorption features in the 220 to 1000 nm range; these are named type I (figure 11, left) and type II (figure 11, right) spectra. Considering chemical composition (table 2) and previous studies (Marfunin, 1984; Lu, 2005), the significant transition metals that contributed to the colors and UV-Vis-NIR spectra of the Guangxi black nephrite were iron and manganese. In both types of spectra, there are two broad bands centered at 726–737 nm and 916–935 nm, which are due to Fe2+↔Fe3+ intervalence charge transfer and combinations of Fe2+ and Fe3+ electron transition, respectively. The strong absorptions in the blue-ultraviolet range with a maximum at 440–460 nm for type I spectra and 355–405 nm for type II spectra are both due to O2–↔Fe3+ charge transfer. In type II spectra, shoulders at 445 nm and 625–635 nm are due to Fe3+/Mn2+ and Fe3+ electron transition, respectively. Absorptions mentioned above result in a transmission centered at 628–645 nm or 515–553 nm in the visible range, which leads to a greenish yellow/brownish yellow or pale green/yellowish green/green bodycolor, respectively.
However, there is no obvious difference in transition metal contents between the samples with two types of color. We infer that differences in their UV-Vis-NIR spectra result from different modes of occurrence of iron and manganese in black nephrite.
DISCUSSION
Iron’s Influence on RI, SG, and Vibrational Spectra. The samples from Guangxi have an iron content (11.67–25.75 wt.% FeO) that is higher than that of white nephrite (0.07–1.09 wt.% FeO, four deposits; Siqin et al., 2012), green nephrite (0.12–4.93 wt.% FeO, nine deposits; Siqin et al., 2012), and even other black nephrite (4.11–14.39 % FeO, Xinjiang; Liu et al., 2011a). For that reason, they have a higher RI (1.625–1.650) and SG (3.015–3.405) than any nephrite previously reported (see Zhang, 2006). Likewise, significant substitution of iron for magnesium indicates that black nephrite from Guangxi is primarily composed of actinolite or ferro-actinolite instead of tremolite. Figure 12 shows that the samples’ RI and SG values increase with iron content. Due to a large amount of minor minerals included in some samples (see table 1), related data can deviate from the normal trend. For sample GB-04, the relatively high RI and SG are believed to result from the diopside content. The relatively low RI and SG of GB-05 are likely caused by its stilpnomelane content. The highest SG, in sample GB-08, is probably related to Fe sulfides in addition to a high iron content.
As seen in figure 13, the mid-infrared, near-infrared, and Raman spectra of the black nephrite are characterized by four M3-OH vibration bands in the 3700 to 3600 cm–1 or 7240 to 7020 cm–1 range. In the crystal structure of calcic amphiboles, each hydroxyl group is coordinated to three neighboring metal ions at two M1 positions and one M3 position, forming a pseudo-trigonal symmetrical (M1M1M3)OH group. M1 and M3 positions are occupied mainly by Mg2+ or Fe2+, forming a perfect isomorphous substitution. For white nephrite composed of tremolite nearly free of iron, there exists only one sharp (Mg)3OH band. For greenish white and green nephrite, bands related to (Mg2Fe2+)OH and (MgFe2+2)OH gradually appear as iron content increases (Burns and Strens, 1966; Feng et al., 2017). For the black nephrite from Guangxi, M3-OH vibration bands are split into four bands due to high iron content. According to Burns and Strens (1966) and Lu (2005), four bands in the mid-infrared and Raman spectra near 3681/3674, 3664/3661, 3646/3643, and 3625/3624 cm–1 are respectively attributed to (Mg)3OH, (Mg2Fe2+)OH, (MgFe2+2)OH, and (Fe2+)3OH fundamental vibrations. Four bands in the near-infrared spectra near 7185, 7156, 7117, and 7077 cm–1 are respectively attributed to the first overtone of (Mg)3OH, (Mg2Fe2+)OH, (MgFe2+2)OH, and (Fe2+)3OH fundamental vibrations (table 4).
For sample GB-04, with the lowest iron content—11.67 wt.% FeO, and an Mg/(Mg + Fe2+) ratio of 0.765)—the relative intensity of the M3-OH bands is (Mg2Fe2+)OH > (Mg)3OH > (MgFe2+2)OH > (Fe2+)3OH. The (Fe2+)3OH band is very weak (figure 13). For GB-10, GB-06, and GB-12, with increasing iron content, (MgFe2+2)OH and (Fe2+)3OH bands are increasingly stronger as they are more closely related to Fe2+, while (Mg2Fe2+)OH and (Mg)3OH bands are increasingly weaker. For sample GB-09, with the highest iron content—25.75 wt.% FeO, and an Mg/(Mg+Fe2+) ratio of 0.343—the relative intensity is (Fe2+)3OH≈(MgFe2+2)OH > (Mg2Fe2+)OH > (Mg)3OH, and the (Mg)3OH band is very weak. Therefore, iron content and Mg2+-Fe2+ isomorphous substitution affect the relative intensity of M3-OH vibration bands, in addition to their number and frequency.
There are also differences in the Raman spectra of the black nephrite samples in the 1400–100 cm–1 range (figure 10, right). For instance, with increasing iron content, the strongest peak at 669 cm–1 gradually shifts toward the lower frequency to 665 cm–1. The relative intensity of the 1060 cm–1/1047 cm–1 peaks becomes stronger, while the peaks at nearly 946 and 408 cm–1 become weaker. And in mid-infrared spectra (figure 8, right), the intensity of the band at approximately 1012 cm–1 becomes weaker with increasing iron content.
Genesis of Black Nephrite. Located at the boundary zone between diabase and limestone bearing siliceous rock, Guangxi black nephrite is a product of contact metasomatism3 occurring after basic magma intrusion into carbonate rock. Therefore, this deposit is of contact metasomatic and skarn origin. Skarn deposits can generally be grouped into Ca-, Mg-, and Mn-skarn by their mineral assemblages. Typical Mg-skarn nephrite from Xinjiang, which is related to dolomitic marbles rich in magnesium (29.61–30.76 wt.% CaO, 22.08–22.52 wt.% MgO, and a Ca/Mg ratio of 0.94–1.01; Liu et al., 2011b), contains Mg-bearing minerals such as olivine, spinel, phlogopite, serpentine, and talc (Tang et al., 1994; Liu et al., 2015). Low-magnesium marbles (54.19 wt.% CaO, 0.21 wt.% MgO, and a Ca/Mg ratio of 183) found in the deposit and the presence of calcic minerals such as actinolite/ferro-actinolite, andradite, diopside, and epidote in the samples (figure 7 and table 3) strongly suggest that the Guangxi deposit is a Ca-skarn deposit. The skarn appears to be spatially zoned as the Xinjiang deposit, with different minerals distributed at different distances from the intrusive rock (figure 4). Moreover, it is remarkable that stilpnomelane, a minor mineral in the black nephrite from Guangxi, can be used as a fingerprint, as it has not been reported to exist in other nephrite deposits.
The Fe2+/(Mg + Fe2+) ratio of actinolite/ferro-actinolite in black nephrite from Guangxi (0.235–0.657) is much higher than that of tremolite-actinolite in D-type (0.001–0.074) and S-type (0.064–0.118) nephrite (Siqin et al., 2012), which is determined by diabase related to its formation. From a chemical composition perspective, basic diabase is richer in iron and magnesium and poorer in silicon (12.00–15.50 wt.% FeO, 3.02–6.59 wt.% MgO, and 45.90–48.30 wt.% SiO2; Zhang and Xiao, 2014), compared to intermediate-acid diorite/granodiorite (2.68–6.16 wt.% FeO, 2.27–4.39 wt.% MgO, and 50.57–58.62 wt.% SiO2; Tang et al., 1994). Hence, diabase and siliceous rock in limestone are likely sources of magnesium and silicon, respectively, whereas the limestone itself is the source of calcium. Rare earth elements (REE) with patterns more similar to limestone than diabase (Wang et al., 2014) suggest that the Guangxi black nephrite formed by the replacement of limestone. In addition, hydrous minerals such as actinolite/ferro-actinolite, stilpnomelane, and epidote formed in the metasomatism stage indicate that fluids were also involved. Therefore, it is proposed that actinolite/ferro-actinolite in black nephrite from Guangxi formed by a reaction between limestone bearing siliceous rock and aqueous solutions bearing iron, magnesium, and silicon:
2CaCO3 + 8SiO2 + xFeO+ (5–x)MgO +H2O → |
Ca2(Fex,Mg5-x)Si8O22(OH)2 + 2CO2 |
The rocks involved in black nephrite formation are limestone bearing siliceous rock and diabase. These are similar to the rocks in the Guizhou deposit. Considering similarities in geographical and tectonic locations and orebody characteristics, we believe that Guangxi and Guizhou represent another large-scale nephrite formation belt in China in addition to the east-west Kunlun Mountains.
CONCLUSIONS
With a pure color and delicate texture, carvings of black nephrite jade from Guangxi in southern China (figure 14) have been favorably received in the market since mining began in 2012. The material is composed mainly of fine-grained and felted actinolite or ferro-actinolite, with minor minerals stilpnomelane, andradite, apatite, epidote, quartz, diopside, pyrrhotite, and pyrite. An abundant iron content—11.67–25.75 wt.% Fe oxides and an Mg/(Mg + Fe2+) ratio of 0.765–0.343—is the main reason for its high refractive index (1.625–1.650) and specific gravity (3.015–3.405). High iron content is also the reason for the black color in natural light for samples with a normal thickness (1.10–6.13 mm) and the greenish yellow/brownish yellow or pale green/yellowish green/green color in transmitted light for thinner samples (0.73–0.97 mm). Moreover, significant isomorphous substitution of Fe2+ for Mg2+ at M1 and M3 positions in the crystal structure of actinolite or ferro-actinolite is responsible for bands with different intensities appearing at approximately 3681/3674, 3664/3661, 3646/3643, and 3625/3624 cm–1 in the mid-infrared/Raman spectra, as well as bands at approximately 7185, 7156, 7117, and 7077 cm–1 in the near-infrared spectra. These are respectively related to (Mg)3OH, (Mg2Fe2+)OH, (MgFe2+2)OH, and (Fe2+)3OH fundamental vibrations as well as their first overtones. As a product of Ca-skarn metasomatism occurring at the contact zone between diabase intrusions and limestone bearing siliceous rock, Guangxi black nephrite originated from source rocks similar to those of Guizhou. It is proposed that the Guangxi and Guizhou nephrite deposits are part of a large-scale jade formation belt in southern China.
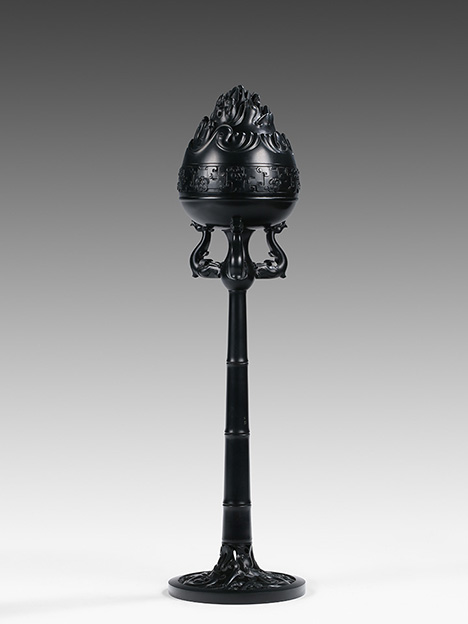
FOOTNOTES |
↩ Back to article ↩ Back to article ↩ Back to article |