Natural-Color Blue, Gray, and Violet Diamonds: Allure of the Deep

ABSTRACT
Natural-color blue diamonds are among the rarest and most valuable gemstones. Gray and violet diamonds are also included here, as these diamonds can coexist on a color continuum with blue diamonds. More so than most other fancy colors, many diamonds in this color range are sourced from specific locations—the Cullinan mine in South Africa and the Argyle mine in Australia. Although blue color is often associated with boron impurities, the color of diamonds in this range (including gray and violet) also originates from simple structural defects produced by radiation exposure or from more complex defects involving hydrogen. These different mechanisms can be characterized by absorption and luminescence spectroscopy. A fourth mechanism—micro-inclusions of grayish clouds or tiny graphite particles in gray diamonds—can be distinguished through microscopy. In this article, we summarize prior research as well as collected data such as color and carat weight on more than 15,000 naturally colored blue/gray/violet diamonds from the GIA database (along with an analysis of spectroscopic data on a subset of 500 randomly selected samples) to provide an unprecedented description of these beautiful gemstones.
INTRODUCTION
Diamond in its pure form would be an uncomplicated material to study scientifically: a perfectly assembled carbon crystal with no color, no structural imperfections or defects at the atomic lattice level, and no inclusions. But no diamond is perfect, and it is these structural imperfections that can create beautiful colors and provide evidence of the growth history of the diamond. Nature has gifted us with diamonds that span the entire color spectrum. In this second in a series of articles about color in natural diamonds (see Breeding et al., 2018 for a discussion of the green color range), we will summarize the causes of blue/gray/violet hues (figure 1), and supplement information in the published literature by providing a compilation of results drawn from the GIA database.
Among fancy-color diamonds, there is greater awareness of blue diamonds because of the prominent display of the Hope diamond at the Smithsonian Institution in Washington, DC—there is no corresponding public stage for other large colored diamonds. This year, 2018, marks the sixtieth anniversary—the diamond anniversary—of the Hope’s permanent exhibition at the Smithsonian. Several other historical blue diamonds are also steeped in legend and royalty (see, e.g., Gaillou et al., 2010). Additionally, recently discovered stones (e.g., Gaillou et al., 2014) capture princely sums at auctions today (see box A). For example, the 12.03 ct Blue Moon of Josephine sold for $48.5 million in 2015 (setting a world record of over $4 million per carat; Sotheby’s, 2015).
During the last decade, GIA has examined over 15,000 naturally colored blue/gray/violet diamonds, including more than 6,000 with unmodified blue color descriptions (figure 2). While there are a few different causes of blue color in diamond, the most familiar cause, accounting for more than half of the natural unmodified blue diamonds seen at GIA, is measurable quantities of boron accompanied by extremely low levels of nitrogen. The Hope diamond, arguably the most famous colored diamond in the world, derives its color from 360 ppb of neutrally charged boron (Gaillou et al., 2010).
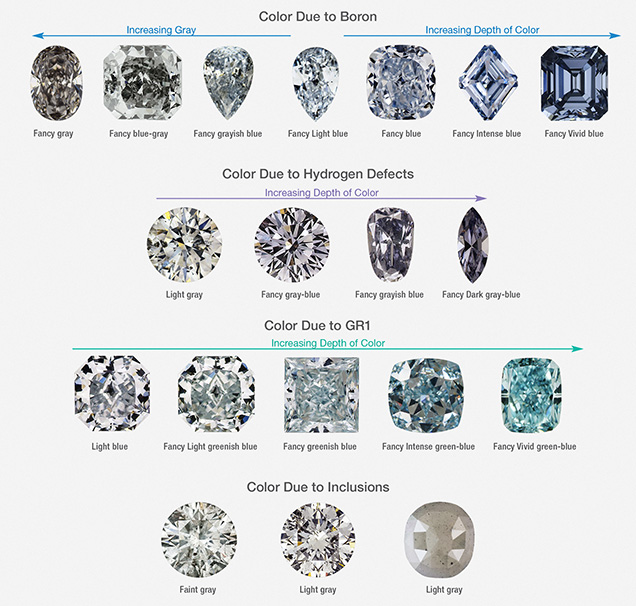
Numerous aspects of gem-quality diamonds in this color range have previously been addressed. Type IIb diamonds were discussed by King et al. (1998), Eaton-Magaña et al. (2008), and Gaillou et al. (2012); hydrogen-defect blue/gray/violet diamonds were explored by Fritsch and Scarratt (1992), Iakoubovskii and Adriaenssens (2002), Fritsch et al. (2007), and van der Bogert et al. (2009). The purpose of this article is to provide a detailed account of the gemological and spectroscopic characteristics of natural blue/gray/violet diamonds colored by various mechanisms in order to help the trade better understand these beautiful and often extremely valuable gems. The description of these diamonds will begin with a discussion of their coloration. As a caveat, we wish to mention that in this series of articles, a good deal of discussion is based on the graded color description of the diamonds (for example, “blue” diamonds are separated from “green-blue” diamonds in later sections and in figures). In the description of color grades, we do not explicitly address that it is more difficult for the eye to interpret subtle hue differences for very light-colored diamonds than it is for more saturated diamonds (King et al., 1994; King, 2006). Therefore, for the lightest color grades, fewer hue names are used and the color description spans a wider portion of the hue wheel. Of the 6,000+ diamonds with unmodified blue color descriptions in this study, 42% were from the lightest color grades (Faint, Very Light, Light; figure 3, right).
CAUSES OF COLOR
Blue/gray/violet color in gem diamonds can span a wide range in the GIA color description terminology (figure 2). For the purposes of this article, we include all natural fancy-color diamonds with blue, gray, or violet as the dominant color (i.e., the final name in the color description). Of the full dataset of 15,000+ blue/gray/violet diamonds seen at GIA over the last decade, most were unmodified blue (37.8%) or gray (33.1%). Next in abundance were those of gray-blue to blue-gray coloration, followed by green-blue diamonds. The lowest percentages belonged to those with violet coloration (figure 3, left). Below we chronicle the main causes of color; three are due to the presence of structural defects and one due to the presence of micro-inclusions. Representative diamonds and visible/near-infrared (Vis-NIR) spectra for these four causes of color are shown in figures 2 and 4, respectively.
Boron. Natural type IIb diamonds were studied in the 1950s and ’60s (Dean, 1965), and aluminum was originally identified as the impurity responsible for the color (e.g., Crowther et al., 1967). Ultimately, boron was unambiguously identified as the natural dopant causing the observed color and semi-conductivity (Collins and Williams, 1971).
Type IIb diamonds are identified by a characteristic infrared absorption feature at 2800 cm–1. The area under this absorption peak can also be used to calculate the uncompensated boron concentration (Fisher et al., 2009; Collins, 2010), which is the total boron concentration minus any donors such as nitrogen. Compensation occurs when electrical acceptors (such as boron in diamond) and electrical donors (such as nitrogen) cancel out each other’s electrical charge. It is this uncompensated boron absorption peak centered at 2800 cm–1 in the infrared region that creates the gradually decreasing absorption gradient that extends into the near-infrared and red areas of the visible spectrum and creates the blue coloration (figure 4A and figure 5). In addition to the absorption that boron creates in the red region of the visible spectrum, plastic deformation–related defects of uncertain structure produce absorption at wavelengths less than 500 nm that can add a gray component (Collins et al., 2000). However, higher boron concentration is generally associated with a higher absorption gradient and a stronger blue color (see, e.g., box A in King et al., 1998 and figure 5). Boron content will not create blue color if sufficient competing defects are also present within the diamond. The necessity of both the presence of boron and the absence of detectable nitrogen—among other donors such as irradiation-related defects—creates the (extremely rare) geological conditions that produce natural type IIb diamonds.
Hydrogen. The observed color in hydrogen-defect diamonds results from the contributions of two broad absorption bands creating transmission windows in the blue and red regions of the absorption spectrum (figure 4B; Fritsch et al., 2007; van der Bogert et al., 2009). Two primary broad absorption bands span the range of 500–760 nm (and generally centered at around 530 and 720 nm, though the central positions can vary between diamonds; see figure 4B), along with a characteristic “hydrogen band” at 835 nm. The relative intensity of these two bands creates transmission windows (blue or red) that determine if the resultant color appears more blue or violet (Fritsch et al., 2007; van der Bogert et al., 2009).
The cause of these broad bands, along with the commonly observed narrow feature at 551 nm, is unknown. The possible relationship between the band at approximately 530 nm in blue/gray/violet diamonds and the 550 nm band in pink diamonds is also still a matter of scientific debate (Iakoubovskii and Adriaenssens, 2002; van der Bogert et al., 2009) despite the close proximity in wavelength position and comparable band width, and the fact that diamonds of both colors are predominantly sourced from Australia’s Argyle mine. Van der Bogert et al. (2009) determined that the band at approximately 530 nm is likely a composite of several independent peaks, and that there are several other gemological distinctions between pink and blue/gray/violet diamonds.
The best-known hydrogen-related defect in natural diamond is the 3107 cm–1 infrared absorption—recently attributed to a hydrogen atom associated with three nitrogen atoms and a vacancy (i.e., a vacant carbon atom position in the lattice; Goss et al., 2014). While the 3107 cm–1 peak in the infrared region has been correlated with the hydrogen-related band at 835 nm, the precise mechanism causing the visible coloration is still unknown (Fritsch et al., 2007). There are certainly other as yet unidentified structural defects involved with the diamond coloration. The presence of hydrogen defects in the diamond structure has been known and studied for decades, but very little is known about how the hydrogen impurities affect diamond color. Nevertheless, high hydrogen concentrations have been determined as a necessary condition for the diamonds in this color range (Iakoubovskii and Adriaenssens, 2002).
In Breeding et al. (2018), we discussed hydrogen defects that occasionally create a green color component. Figure 6 shows a distinction between hydrogen-related spectra obtained for green diamo-nds (upper set) and those for violet-gray diamonds (lower set). Both have a pronounced band at approximately 835 nm. This hydrogen band occurs in both green and blue/gray/violet diamonds containing high quantities of hydrogen, and it was described by Fritsch et al. (2007) as a triple band including absorptions at 829, 837, and 844 nm. However, the green diamonds have much less pronounced bands at 530 and 720 nm, along with more pronounced “cape” features (e.g., N2 at 477.6 nm and N3 peaks at 415.2 nm). In green diamonds, the greater absorption at low wavelengths (below 500 nm), plus a comparatively minor contribution from the 530 and 720 nm bands, shifts the transmission window into the green region of the visible spectrum.
The two absorption bands in these diamonds create two transmission windows (again, see figure 6). This can result in a color shift under two different light sources. This phenomenon is most associated with alexandrite, and occasionally seen in natural diamonds (Liu et al., 1998) and treated diamonds (Eaton-Magaña, 2016). Many of these hydrogen-defect diamonds receive color grades within the gray-to-blue range when viewed in the GIA color-grading environment (King et al., 1994), but they can often appear violet under other light sources (see figures 2 and 7). Although very few diamonds receive a color grade with a violet component (again, see figure 3, left), there are likely many more diamonds with this cause of color in which violet can be observed under incandescent lighting conditions.
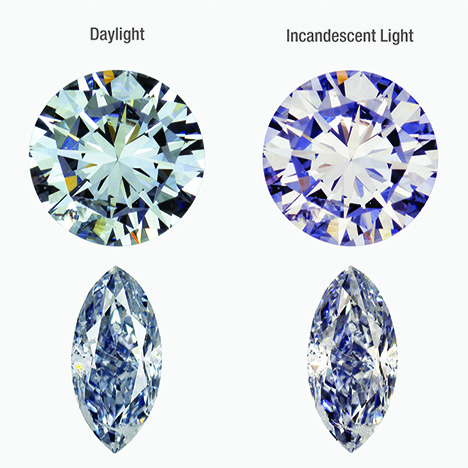
GR1. As discussed extensively in Breeding et al. (2018), GR1 (general radiation 1) defects are empty or vacant lattice positions in the diamond structure, and they serve as the most common mechanism for green to green-blue color in gem diamonds (Shigley and Fritsch, 1990; Collins, 2001; Shigley and Breeding, 2013). Vacancies are created when radiation (both natural and laboratory-induced) provides sufficient energy to displace carbon atoms from their normal positions in the diamond lattice. GR1 vacancies cause the diamond to absorb light in the red part of the visible spectrum (zero phonon line [ZPL] at 741 nm with associated broad absorption extending from approximately 550 to 750 nm; see figure 4C).
Figure 8 compares the Vis-NIR absorption spectra of a blue, a green-blue, and a green diamond. Generally, green diamonds have a greater absorption at lower wavelengths in the UV to blue region of the spectrum (below 500 nm), which shifts the transmission window into the green portion of the visible spectrum. This increased absorption is mostly associated with nitrogen-related defects such as N2 and N3 (Breeding et al., 2018). As naturally irradiated diamonds often have high quantities of these nitrogen-related defects, most of them are green. Green-blue to blue diamonds often have lower nitrogen-related absorption in the UV to blue region (top two spectra in figure 8), and many are type IIa.
As with green diamonds, jewelers must take great care during jewelry repair processes when heating blue diamonds colored by this mechanism in order to avoid destroying the GR1 defects and losing the blue color.
Inclusions. The micro-inclusions and cloud-like features that cause gray color in diamonds (e.g., figure 9A and 9B)1 should not be confused with the micro-inclusions often seen in Fancy white diamonds (which are almost always type IaB). The micro-inclusions in Fancy white diamonds provide an opalescent appearance (Moses and Reinitz, 2000; Gu and Wang, 2017).
1Since a variety of hues can appear “gray” at very low color saturation, these diamonds have a variety of subtle color sensations and can appear, for example, as either more bluish or more yellowish and still be graded as the color gray (King et al., 1994, King, 2006). Many of the diamonds that derive their color from inclusions also show a typical cape spectrum (e.g., figure 4D), which can often impart a yellowish (or warm) appearance to these gray diamonds. In contrast, many gray diamonds due to boron impurities will have a bluish (or cool) appearance. The color sensation is not sufficient to affect the color grade but can lead to variations in appearance among diamonds with the same color description.

In contrast, these gray diamonds colored by internal features are typically type IaA±B (determined as type IaA, IaAB, or IaB) or saturated (where the aggregated nitrogen absorption is greater than the detection limits of infrared spectroscopy, generally greater than 500 ppm) type Ia. The observed particle clouds appear whitish to grayish. Except for nitrogen impurities, the Vis-NIR spectrum contains a decreasing absorption gradient toward higher wavelengths (figure 4D). These clouds are sometimes termed “hydrogen clouds” due to the elevated intensity of the 3107 cm–1 infrared peak within these areas. However, there is little similarity between these so-called hydrogen-cloud gray diamonds and the hydrogen-related blue-to-violet diamonds discussed previously.
Far more likely is that these gray diamonds are similar to the mixed-habit type IaAB diamonds containing gray/colorless zoning (the term “mixed habit” refers to standard {111} growth accompanied by growth of approximately {100} orientation). These diamonds have been studied extensively due to their simultaneously grown cuboid and octahedral sectors (e.g., Smit et al., 2016). Within the cuboid sectors are many micro-inclusions that have been identified as graphite (Smit et al., 2016; Eaton-Magaña et al., 2016), and those sectors often have a grayish appearance. Analysis of the micro-inclusions within polished flat-plate samples indicated that the individual inclusions are quite small—no more than 1 μm. When those samples were heated to 1200°C, they were still gray, but the graphitic inclusions were enlarged to 3–4 μm and appeared dark in the microscope (Eaton-Magaña et al., 2016). This indicates that both the small gray micro-inclusions and the larger, darker micro-inclusions impart a gray bodycolor. Photomicrographs obtained from flat plates of a natural mixed-habit diamond show grayish clouds and graphitic inclusions (figure 9, C–E), and a natural diamond shows noticeable graphitic inclusions (figure 9F). Although the micro-inclusions in several mixed-habit research diamonds have been identified as graphite (e.g., Smit et al., 2016), and likely a good number of the gray diamonds colored by inclusions are due to graphite micro-inclusions, we should not presume that all micro-inclusion and cloud observations within this color category are due to graphite.
OCCURRENCE AND FORMATION
The formation mechanism for blue/gray/violet diamonds in the earth varies significantly depending on the defects responsible for the color. Stones colored by micro-inclusions or by hydrogen- or boron-related defects incorporate these impurities, along with any nitrogen impurities, when they crystallized deep in Earth’s mantle. Long residence times at high temperatures and pressures contribute to the final arrangement of structural defects within the diamonds.
The precise formation mechanism that leads to type IIb diamond—measurable boron concentrations accompanied by extremely low nitrogen levels—is still a matter requiring further study. It is unclear what distinguishes the Cullinan mine from other mines and makes it a reliable source for type IIb diamonds; however, recent evidence demonstrates that these are “superdeep” diamonds originating at sublithospheric mantle depths (> 600 km) and that the boron is likely sourced from subducted oceanic lithosphere (Smith et al., 2018). Similarly, the blue/gray/violet diamonds colored by hydrogen defects are almost exclusively from the Argyle mine. Diamonds colored by GR1 defects are generally thought to obtain their color from late-stage interactions with radioactive minerals or fluids in the earth’s crust over thousands to millions of years (see Breeding et al., 2018, and references therein). Since elevated temperatures will change their color, this natural irradiation likely occurred in the earth’s crust, relatively close to the surface.
The blue/gray/violet diamonds colored by hydrogen-related defects, as well as the gray diamonds colored by inclusions, likely underwent more rapid growth than typical diamond growth, with processes similar to the cuboid growth observed in mixed-habit diamonds (Fritsch et al., 2007; Smit et al., 2016). This faster growth rate would allow the growing diamonds to trap impurity atoms, such as hydrogen and nickel, at much higher concentrations (see the “Luminescence” section below; Fritsch et al., 2007). Similarly, this fast growth model applies to the gray diamonds colored by inclusions, as it allows the diamond to trap high quantities of mineral and fluid inclusions (Smit et al., 2016).
LABORATORY GRADING
Stones weighing up to 1.0 ct comprised 63% of the 15,000+ natural blue/gray/violet diamonds examined at GIA (see figure 10). Noticeable spikes in quantity are observed near important carat-weight thresholds (e.g., 0.5, 1.0, 1.5, and 2.0 ct). This chart shows that faceting and polishing decisions may maximize the face-up color and appearance, but weight thresholds are often important considerations as well. The diamonds in figure 10 are shown according to their general color groups. The data indicate that the diamonds with gray-violet, green-blue, and gray-blue hues are much more prevalent below 1 carat than at higher weights. Above a carat, most are blue or gray.
Although fancy-color diamonds are often fashioned as fancy shapes to enhance their face-up color, round brilliants represent a plurality (44%) of the 15,000+ diamonds (figure 11, left), followed by pears (16%), rectangles (9%), and cushions (8%). The majority of diamonds with pale color (i.e., Faint to Fancy Light) are faceted as rounds (figure 11, right), whereas fancy shapes are dominated by the deeper colors (Fancy to Fancy Dark). Those faceted as round brilliants were likely interpreted as D to Z material during the pre-faceting steps.
To determine the distribution of the four major causes of color, we further analyzed the Vis-NIR and infrared (IR) absorption spectra of a representative sampling of 500 natural blue/gray/violet diamonds (randomly selected from the 15,000+ in our GIA database) and identified the cause of color for each. This subset of data was used to generate figures 12 and 13. For this subset, we observed that 177 (35%) of natural blue/gray/violet diamonds were colored by boron and 132 (27%) by the GR1 defect, 152 (30%) by hydrogen defects, and 37 (7%) by inclusions (figure 12, top). We also determined the boron concentration of the 177 type IIb diamonds (Fisher et al., 2009; Collins, 2010), and the nitrogen aggregate concentration in 48 unsaturated type IaA±B diamonds (Boyd et al., 1994, 1995). Two other diamonds were colored by the 480 nm band and will be discussed in the “Unusual Examples” section.
The data become more illustrative when separated out into color groups. For example, the green-blue color grade is populated exclusively with diamonds colored by GR1, while inclusion-related defects are found solely within the gray color region. The blue-gray to gray-blue region is populated almost evenly with type IIb (boron) and hydrogen-defect diamonds, with a small minority colored by GR1 defects. The violet category is dominated by H defects but also contains a low amount of type IIb diamonds. The blue category—the most valuable hue within this range—has a majority of diamonds colored by boron, with 37% due to GR1 defects (although these generally have low color saturation).
Figure 12 (bottom) shows the distribution of these color centers based on the color saturation from Faint to Fancy Deep. Diamonds colored by GR1 or H defects are usually found among the lighter hues, while type IIb diamonds dominate among the more saturated color descriptions. The GR1-defect diamonds with Intense or Vivid color grades were all green-blue and not an unmodified blue color.
Figure 12 also illustrates the perceived dominance of type IIb diamonds within the blue/gray/violet color range. They frequently have an unmodified blue color description and more often have intense color than any of the other groups. This combination of saturated color and attractive blue hue commands the highest value, especially at the higher weights in which other colors in this range are not well represented. With the exception of a handful of violet diamonds offered at the Argyle mine’s annual tender (e.g., King et al., 2014; Rio Tinto, 2017), the most famous and notable of the blue/gray/violet diamonds are type IIb.
GEMOLOGICAL OBSERVATIONS
Blue/gray/violet natural diamonds have numerous interesting features that can be observed with standard gemological equipment. These properties, however, are quite variable depending on the cause of the color. In this section, we review the major observations from diamonds colored by each group of defects.
Colored by Boron. Type IIb diamonds often show visible phosphorescence (figure 14A). The most famous example is probably the vibrant red phosphorescence displayed by the Hope diamond (Eaton-Magaña et al., 2008) which can be observed for up to one minute. Regardless of the observed color of phosphorescence, natural type IIb diamonds generally have two well-defined phosphorescence emission bands (figure 14B), centered at 500 nm (blue phosphorescence) and 660 nm (red). Natural diamonds that show only the 500 nm band are predominantly an unmodified blue, while those type IIb diamonds with gray or violet components typically show the 660 nm band (Eaton-Magaña and Lu, 2011; figure 14C).
The generally accepted mechanism for phosphorescence in type IIb diamonds is “donor–acceptor pair recombination” (DAPR), which is reviewed in depth by Dean (1965). In this mechanism, “holes” bound to acceptor atoms and electrons bound to donor atoms are activated by energy from the ultraviolet source, and they subsequently recombine and emit light equivalent to their energy difference (plus an electrostatic correction). Boron has been positively identified as the acceptor in the mechanism (Collins and Williams, 1971). Since gray coloration is largely caused by defects related to plastic deformation, it is highly likely that the donor corresponding to the 660 nm band is also influenced by plastic deformation, while the donor responsible for the 500 nm phosphorescence band is not (Eaton-Magaña and Lu, 2011).
Colored by Hydrogen-Related Defects. As with green diamonds colored by hydrogen defects (Breeding et al., 2018), diamonds in the blue color range also often contain whitish particle clouds with sharp boundaries and relatively non-included regions. As these clouds can create gray color on their own, their presence can influence the grayish coloration of these diamonds. However, the color contribution of inclusions in these diamonds has not been fully evaluated.
When viewed under UV light, many hydrogen-rich diamonds fluoresce yellow. This observation has been consistent for many different colors of diamond, including “chameleon” and green H-defect diamonds (Fritsch et al., 2007; van der Bogert et al., 2009; Breeding et al., 2018). The same observation was true for the 152 blue/gray/violet diamonds colored by hydrogen that were examined for this study—93 (61%) fluoresced yellow under long-wave UV, and 137 (90%) did so under short-wave UV.
Colored by GR1. The most distinctive gemological feature of blue diamonds colored by radiation damage is surficial radiation spots and internal color zonation. Radiation stains occur as either green or brown patches of color on the surface of a rough diamond or within fractures. Polishers will often preserve a portion of these radiation stains after faceting, particularly in the girdle area (again, see figure 11), to provide confirmation that the GR1 defect originated from natural radiation and not laboratory irradiation.
Although the bodycolor is green-blue to blue, the radiation stains are colored green and turn brown if heated above 550–600°C (Nasdala et al., 2013; Eaton-Magaña and Moe, 2016). Radiation stains in these diamonds still appear green (figure 15, left) even when the bodycolor appears blue. In the green radiation stains, optical absorption spectra show GR1-related absorption at high wavelengths and vacancy cluster–related general absorption at lower wavelengths (Nasdala et al., 2013), thus creating a transmission window within the green area. For brown radiation stains, the color is determined solely by the vacancy cluster absorption, as the heating brought about depletion of the GR1 absorption. At low wavelengths, therefore, the absorption within the radiation stains and the absorption within the bulk of the diamond (nitrogen “cape” absorption; figure 8) are brought about by separate causes. If the cape absorption is not present, then the bulk of the diamond appears blue while the radiation stain appears green.
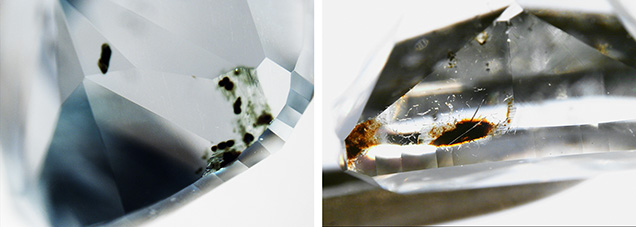
Colored by Inclusions. Gray diamonds colored by internal inclusions have few indicators regarding cause of color within their absorption spectra, so microscopic observation of cloud-like features or graphitic inclusions is essential for these stones. As there are several possible origins for gray color in diamond, a Vis-NIR spectrum with common features (e.g., figure 4D) in conjunction with the presence of cloud-like inclusions establishes the inclusions as the cause of the gray color. Due to the abundance of color-causing inclusions, these diamonds also have very low clarity grades—typically the Included clarity grades (I1 to I3).
ABSORPTION SPECTROSCOPY
Absorption spectroscopy measurements are nondestructive and provide a detailed glimpse of a diamond’s atomic structure by passing light of different wavelengths through the stone and measuring what wavelengths (energies) are absorbed by the impurities and defects present. IR absorption gives information about the impurities present in the diamond lattice, while optical absorption reveals the color-producing defects. All of the atomic defect groups of blue/gray/violet diamonds have unique spectroscopy features. Standard testing conditions and instrumentation are applied to all diamonds submitted to GIA. These methods have been detailed in other publications (e.g., D’Haenens-Johansson et al., 2015) and summarized for this series of articles in Breeding et al. (2018).
IR Absorption. Details of the major impurities present, as well as their configuration relative to the rest of the carbon lattice in diamond (diamond type), are directly and quickly determined by IR spectroscopy (Breeding and Shigley, 2009).
For diamonds in this color range, the cause of color can sometimes be inferred from the diamond type (figure 13). Type IIa diamonds are generally colored by irradiation; type IIb, by definition, contain boron impurities. H-defect diamonds in this color range require high concentrations of hydrogen and therefore generally high nitrogen (Iakoubovskii and Adriaenssens, 2002). Most H-defect diamonds studied here were saturated type Ia diamonds.
Boron. The uncompensated boron concentration for 177 type IIb diamonds was determined from the IR spectra. The values ranged from 25 ppb for a Faint blue diamond up to 1500 ppb for a Fancy Vivid blue. Type IIb diamonds with lower boron concentrations would likely receive a grade in the D to F range (King et al., 2008). The range of uncompensated boron concentrations was greatest for unmodified blue type IIb diamonds (several with values above 500 ppb), while gray type IIb diamonds spanned a much narrower range (only up to 400 ppb) and gray-blue and blue-gray were at intermediate ranges.
The highest value (1500 ppb) and median value (300 ppb) were, unsurprisingly, lower than the highest value and median value observed in HPHT synthetic diamonds (4630 ppb and 650 ppb, respectively; Eaton-Magaña et al., 2017b), as manufacturers are able to deliberately introduce large quantities of boron into the growth chamber. Of the 177 type IIb diamonds examined from the 500 diamond subset, 36% had a color grade of Intense, Vivid, Dark, or Deep. Of the type IIb HPHT synthetics examined in Eaton-Magaña et al. (2017b), 85% had color grades in that range.
Hydrogen Defects. Most of the diamonds colored by hydrogen-related defects had very high concentrations of nitrogen aggregates—the nitrogen-related peaks were saturated within the IR absorption spectra. Generally, there were more than 500 ppm of total nitrogen aggregates for these samples. The diamonds with saturated IR spectra are termed here as “type Ia,” as the spectra do not permit us to further specify A or B aggregates. Therefore, relative concentrations (A or B) and total concentrations could not be determined. Diamonds that are termed “type IaA±B” have a sufficiently low concentration of nitrogen aggregates to allow us to evaluate specific aggregates and concentrations. A small percentage (3%) of diamonds colored by H defects were in this category, and most of these were type IaA>B.
GR1. Diamonds colored by GR1 varied greatly in nitrogen concentration from type IIa (no measurable nitrogen) up through a measurable total nitrogen concentration of several hundred ppm; none of those surveyed from the subset of 500 diamonds were saturated type Ia. About one-third (41 of 132) of the diamonds colored by GR1 were type IIa. Of these, 74% were blue (the remaining were green-blue or a few gray). Among the type IaA±B diamonds, most were green-blue (58%). Nitrogen concentrations were calculated for the IaA±B diamonds colored by GR1, and little correlation with bodycolor was noted; however, the highest total nitrogen concentrations were noted for the green-blue diamonds with Fancy Vivid to Fancy Deep color grades. Most of the diamonds were type IaAB with A
Inclusions. The diamonds colored by inclusions spanned all nitrogen aggregation combinations (type IaA, IaAB, or IaB). In contrast, Fancy white diamonds are almost always type IaB.
Optical Absorption. Visible/near-infrared spectroscopy measures the color center absorptions that produce the color we see in a diamond. In the “Causes of Color” section, we discussed the primary visible absorption features responsible for color in these diamonds due to boron, GR1, and hydrogen-related defects.
The optical absorption spectra for diamonds colored by boron or inclusions generally have minimal usefulness (figures 4A and 4D). Type IIb diamonds show only a slope of decreasing absorption from the IR to UV regions, while diamonds colored by inclusions will typically just show cape-related features (e.g., N2 and N3), which do not explain the gray coloration. In these cases, the optical absorption spectra serve to eliminate other potential causes of color and to provide confirmation of separate gemological observations (e.g., IR absorption, PL spectra, and phosphorescence for type IIb diamonds, as well as microscopy for inclusion-related diamond color).
Hydrogen Defects. For H-rich diamonds, several peaks are commonly observed in addition to the major absorption bands discussed earlier. Most of the unmodified gray diamonds in this category (70%) showed N2/N3 peaks, even in those with pronounced bands at around 530 and 720 nm; however, N2/N3 peaks were seen in very few diamonds with a blue or violet color (figure 4B and figure 6). The 551 nm feature was seen in most diamonds with blue or violet coloration (94%), and in very few gray diamonds (13%). A few diamonds also showed a narrow peak at about 594 nm and weak peaks at 503, 615, 620, 631, and 671 nm.
GR1. Radiation damage in diamond produces a number of defect centers in optical spectra in addition to the primary GR1 color center. The most commonly observed defects occur at 595 and 667 nm. The 595 nm center is a defect of uncertain structure consisting of nitrogen atoms and vacancies and is no longer detected after annealing above 1000°C (figure 4C). The 667 nm center consists of a carbon interstitial (a term used for an atom within the crystal structure that is not positioned at a standard lattice position; Green, 2013; Breeding et al., 2018) that anneals out at 400–500°C (Zaitsev, 2003).
PHOTOLUMINESCENCE SPECTROSCOPY
Photoluminescence (PL) spectroscopy is one of the most sensitive and useful techniques for defect characterization in diamond. This method uses lasers of different wavelengths to produce emission spectra that reveal the defects present in a stone. Defects occurring at concentrations as low as the ppb level can easily be detected using this method (Eaton-Magaña and Breeding, 2016).
Boron. The PL spectra of type IIb diamonds have peaks that are generally not detected in diamonds without boron. These include features at 648.2 nm (identified as boron-interstitial; Green, 2013) and 776.4 nm (tentatively assigned as a boron-vacancy complex; Eaton-Magaña and Ardon, 2016). Additionally, nearly all natural type IIb diamonds have the interstitial-related 3H peak (ZPL at 503.5 nm). Nitrogen-related defects are only rarely observed, and generally only as NV0 at 575 nm (figure 16, top).
Hydrogen Defects. The PL spectra for diamonds colored by hydrogen defects generally show a high number of nickel-related peaks (figure 16, bottom) Iakoubovskii and Adriaenssens, 2002; Fritsch et al., 2007; van der Bogert et al., 2009). Similar Ni-related PL spectra are commonly seen in chameleon diamonds (Hainschwang et al., 2005) and mixed-habit diamonds (Lang et al., 2004; Yelisseyev and Kanda, 2007; Eaton-Magaña et al., 2017a). The nickel-related peaks occur at 523.4, 603, 694, 700, 926, and 948 nm; those with greatest intensity occur at 700 and 926 nm. Although these peaks are ascribed to nickel—and some, such as the 926 nm peak, are proposed as Ni-N defects (e.g., Hainschwang et al., 2005)—the specific composition, charge state, and interrelationships between the various detected peaks are currently unknown.
GR1. PL spectra collected from diamonds colored by GR1 can be quite variable due to irregular distributions of radiation damage across a stone’s surface and interior. PL features related to radiation damage are also dramatically affected by heating, even to relatively low temperatures of a few hundred degrees (Eaton-Magaña and Moe, 2016). The strongest PL peaks in these green-blue to blue diamonds are vacancy-related defects such as GR1 (ZPL at 741.2 nm), interstitial-related peaks such as 3H (503.5 nm), and nitrogen-related defects such as H4 (495.9 nm), H3 (503.2 nm), NV0 (575 nm), and NV– (637 nm).
Inclusions. PL spectra of gray diamonds colored by inclusions can vary depending on whether or not the spectra were collected from an included region. Typically, most PL centers are found to have higher intensity in the gray, inclusion-rich sectors than in the colorless, inclusion-poor sectors (Eaton-Magaña et al., 2017a). These include nitrogen- and/or vacancy-related centers such as S3, H3, 612.4 nm, GR1, and H2, along with the nickel-related centers with peaks at 523.4, 603, 694, 700, 926, and 948 nm. Although these latter peaks are correlated with nickel, the specific compositions are currently unknown. In contrast, the NV– (637 nm) center is stronger in colorless, inclusion-poor sectors.
IDENTIFICATION CONCERNS
Boron. Type IIb diamonds may be subjected to HPHT treatment to reduce the grayish component (comparable to HPHT treatment that reduces the brownish component in type IIa diamonds; Fisher et al., 2009). Blue HPHT synthetic diamonds are commonly produced at large sizes (Moe et al., 2016). However, there are very few difficulties separating natural-color blue diamonds from type IIb treated or synthetic diamonds, and they are reliably distinguished based on DiamondView imaging and/or PL spectroscopy.
GR1. Like their green counterparts, blue diamonds colored by GR1 receive a higher than standard incidence of “Undetermined” color origin reports from gemological laboratories. As with green diamonds colored by GR1, the presence of radiation stains is helpful in establishing a natural origin for irradiated blue diamonds.
Hydrogen Defects. There is no known method of treatment to mimic H-related defects in natural diamonds in this color range. The hydrogen-related peak at 3107 cm–1 in the IR region has been correlated with the optical absorption defects causing these colors. While the 3107 cm–1 peak can be increased with annealing or introduced in synthetic diamonds, those laboratory processes do not create the associated absorption of the hydrogen band at 835 nm in optical absorption spectroscopy or the other features seen in these blue/gray/violet diamonds.
Inclusions. There is no known method of treatment to mimic the micro-inclusions in natural diamonds in this color range. Heating of mixed-habit type IaAB diamonds containing micro-inclusions of graphite causes these inclusions to enlarge; the diamonds become darker gray and ultimately black. The graphite inclusions in such heated black diamonds often have a distinctive hexagonal morphology (Eaton-Magaña et al., 2017a). Low-quality CVD synthetic diamonds can have graphitic inclusions along with pinpoint inclusions resembling clouds; additionally, many have a gray color grade (Eaton-Magaña and Shigley, 2016). But CVD synthetics in this color range are always type IIa with distinctive DiamondView features and PL spectra.
In the last few years, GIA’s laboratory has seen a much higher quantity of these diamonds. When we examined 500 of the blue/gray/violet diamonds in greater detail, 37 of them owed their color to inclusions. Of these, 31 were submitted between 2013 and 2016, with the remaining six submitted from 2008 to 2012.
UNUSUAL EXAMPLES
Less than 1% of the diamonds in the blue/gray/violet range attributed their color to a weak 480 nm band. All of these diamonds were gray. Typically, diamonds with a 480 nm band show chameleon behavior or a yellow-orange coloration. A majority of chameleon diamonds have gray as a color modifier (Breeding et al., 2018). These few gray diamonds are likely on the extremely low end of color saturation for chameleons, and they have insufficient visible absorption features to present any color besides gray. As with the gray diamonds colored by inclusions, these gray chameleon diamonds also tend toward warmer tones instead of the cooler, bluish tones of gray type IIb diamonds.
Generally, diamonds that show a 480 nm band in their optical absorption spectra have strong yellow fluorescence to both long- and short-wave UV radiation and weak yellow phosphorescence to short-wave UV. The gray diamonds with a 480 nm band studied here did as well. Very little is known about the defect causing this band.
OUTLOOK
In this article we have discussed the four major causes of blue/gray/violet color in gem diamonds. Based on the results obtained for the randomly selected subset of 500 diamonds from the 15,000+ diamonds in this color range, these four groups comprise over 99% of the natural-color blue diamonds seen at GIA’s laboratories in the last decade. As with all natural diamonds, these gems act as messengers from deep within the earth. The type IIb diamonds in particular, which include several with large size and saturated hue, have captivated royalty and other gem connoisseurs for centuries.
Although many famous and historical blue diamonds were once found in India, today most type IIb diamonds are sourced from South Africa’s Cullinan mine, and most hydrogen-defect diamonds come from the Argyle mine of Australia. As the Argyle mine is set to close within the next few years and the Cullinan mine is projected to close in 2030 (Petra Diamonds, n.d.), it will be interesting to monitor the future of these blue/gray/violet diamonds. These already rare diamonds may themselves become consigned to legend.