The Extraordinary Backstory of Natural Diamonds: A Diamond Is (and Has Been) Forever
Diamonds have a long history as a premier gemstone—a natural consequence of their beauty, rarity, and superlative physical properties such as extreme hardness. Diamonds that are mined for use as gemstones have an innate appeal and air of luxury. In ancient Rome, Pliny the Elder called diamond “the most valuable, not only of precious stones, but of all things in this world.” The utility of diamond as a tool for cutting is also prized. It has even found niches in modern high-tech applications. However, our love affair with this transparent crystalline form of carbon is virtually blind to its geological backstory, arguably one of its most compelling facets.
In the decades since GIA introduced the Four Cs quality-grading concept for polished diamonds in the 1940s, scientists’ understanding of where, when, and how diamonds form has changed radically. They form deeper underground than virtually anything else you can touch. They are more ancient than initially thought, with some dating back to 3.5–3.3 billion years ago (three-quarters of the earth’s age). Diamonds preserve evidence over both space and time of this ancient geologic origin in the form of pristine inclusions of minerals and geological fluids. Processes that contribute to diamond growth involve the movement of rocks and fluids inside the earth at a scale of hundreds of kilometers, a testament to the fact that our planet is dynamic and evolving rather than a static ball of rock. Much of this activity is attributable to plate tectonics and subduction, which is the slow sinking of oceanic plates into the mantle.
In a sense, geologists are beginning to unravel a fascinating new identity for diamonds, giving Pliny even more reason to have admired them. They have evolved from a curiosity to something of great scientific value and excitement. However, public awareness of this development has lagged. Here we review the story of diamond formation alongside other aspects of natural diamonds and their extraction from the earth, showcasing them in a new light.
DIAMONDS ARE DEEP: CARBON IN THE MANTLE
At the earth’s surface, graphite is the stable crystalline form of carbon, with a density of 2.26 g/cm3. Diamond is a much more compact form of carbon, with a density of 3.52 g/cm3, 56% higher than the density of graphite. Great confining pressure is required to stabilize the denser configuration of carbon atoms and crystallize diamond, which is why it forms deep in the mantle. Once formed, diamond remains in that form of carbon under all conditions that normally exist at the earth’s surface.
The physical and chemical conditions of diamond formation have largely been constrained by studies of their mineral inclusions. Most diamonds crystallize within the base of old and thick parts of continents, called cratons, at depths of 150–200 km and temperatures of 900°–1300°C (Stachel and Harris, 2008). These are known as cratonic or lithospheric diamonds. Some rarer diamonds form at depths as deep as 200–800 km, below the lithosphere, and are called sublithospheric or superdeep diamonds (Shirey et al., 2024). Some unusual kinds of mineral inclusions are contained in sublithospheric diamonds, and these serve as the basis to constrain their depth of formation. Figure 1 illustrates just how vast these depths are. Virtually all the rocks and minerals observable at the earth’s surface, including most gemstones, form at relatively shallow depths within the continental crust. In contrast, diamonds are easily the most deeply derived things humans can touch. Much like meteorites from space, they are “alien” materials from deep underground and were only brought to the earth’s surface by an unusual set of geologic circumstances.
Although diamonds originate very deep in the earth, when carried up by kimberlites or related volcanic eruptions, they can endure the upward journey because it is quick and survive indefinitely at the earth’s surface because of their hardness, strength, and chemical robustness. Inclusions trapped inside diamonds can survive as well, being protected and shielded by their host. This makes inclusions in diamonds the most deeply derived well-preserved samples of the mantle. These inclusions can be not only minerals but also fluids or gases trapped at high pressures.
The range of possible residual pressures retained by some inclusions is nicely demonstrated by rare inclusions of nitrogen in either liquid or solid form, the latter having a pressure of 10.9 Gpa (Smith et al., 2013; Navon et al., 2017). For comparison, 1 gigapascal is about 10,000 times atmospheric pressure at sea level or about 10 times the pressure at the deepest part of the Mariana Trench in the Pacific Ocean.
Possibly the highest recorded pressure inside an inclusion (for a diamond above the earth’s surface) is that of a phase called ice-VII, with a reported pressure of 24 GPa, equivalent to the ambient pressure at the bottom of the mantle transition zone (Tschauner et al., 2018). Inclusions from both the lithospheric and sublithospheric mantle are invaluable samples for scientific study because they are the only direct source of mantle information. They speak not only to diamond formation but also to the broader structure, composition, and processes of the mantle.
A more recent discovery based on inclusions has shown that some of the largest and highest-quality gem diamonds are derived from the sublithospheric mantle. Specifically, type IIb (boron-bearing) and so-called CLIPPIR (Cullinan-like, large, inclusion-poor, pure, irregular, and resorbed) diamonds have been found to contain high-pressure mineral assemblages indicating formation at depths between 360 and 800 km (Smith et al., 2016, 2017, 2018). Famous gem diamonds within these “superdeep” categories include the Hope, the Koh-i-Noor, the Lesedi La Rona, and the Cullinan. The faceted gems cut from the 3,106 ct Cullinan have been a cherished centerpiece of the British Crown Jewels for more than a century, based on their exquisite natural beauty and rarity. Now these diamonds have returned to the limelight, including coverage from the BBC (Gorvett, 2022), for their extreme depth of origin.
DIAMONDS ARE ANCIENT
When De Beers introduced the slogan “A diamond is forever” in 1947, they were referring to diamond as a symbol of everlasting love. But four decades later, when the first measurements of diamond ages showed some to be more than three billion years old (Richardson et al., 1984), the romantic tagline became even more fitting. Prior to these measurements, there was considerable debate over whether the diamonds brought up in kimberlite magmas were phenocrysts (crystals grown directly from the magma) or xenocrysts (foreign, preexisting crystals simply picked up by the magma). The latter proved true—the three-billion-year-old diamonds must have grown and lain dormant in the mantle for billions of years before being swept to the surface in the Cretaceous-age (145–66 million years, or Ma) Kimberley and Finsch kimberlites.
Diamond growth may occur somewhat quickly compared to its age, building up a crystal layer by layer over thousands or perhaps millions of years. The subsequent period of mantle residence at conditions of high pressure and temperature can permit changes in the diamond morphology, internal structure, and atomic-scale defects. A well-known change affects the configuration of nitrogen atoms, the most abundant impurity in natural diamonds. Nitrogen atoms migrate from their isolated initial configurations to form pairs, and slowly the pairs reconfigure into groups of four atoms plus a vacancy. Taken collectively, these changes create the ancient geologic signature of natural diamonds, which cannot be created during very brief laboratory growth.
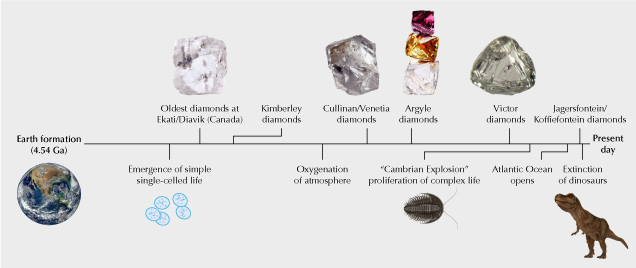
As shown in figure 2, diamonds have formed over a wide range of the earth’s geologic history. The oldest dated examples are diamonds with an age of 3.5–3.3 Ga (billion years) from the Diavik and Ekati mines in northern Canada. For perspective, the age of the earth is 4.54 Ga. Many diamonds are ancient and predate the so-called Cambrian Explosion at 541 Ma, when a prolific increase in the complexity and abundance of life appears in the fossil record, one of the most significant time stamps in geology. However, not all diamonds fall within the Precambrian span of distant geologic time. Some fairly young diamond ages around 90 Ma have been measured at Jagersfontein and Koffiefontein in South Africa. Most deposits have multiple diamond populations that can vary in age. Jwaneng, Letlhakane, and Orapa in Botswana each contain six or more dated episodes of diamond formation (Smit et al., 2022).
It is also worth noting that the majority of fibrous diamonds, a non-gem diamond variety with abundant micro-inclusions that give them a translucent or opaque appearance, are understood to be close in age to their kimberlite hosts and therefore relatively young. It is even possible that diamonds are forming right now and may be carried to the surface by some future kimberlite millions of years from now. A recent review of diamond dating and ages is given by Smit et al. (2022).
The fact that diamonds can be dated is exciting, but the process is far from straightforward. Strictly speaking, the ages described here are mineral inclusion ages. The interpretation is that the age corresponds to how long the mineral has been trapped and sealed within the host diamond, thereby giving the age of diamond formation. Dating is based on exploiting radioactive elements in some inclusions that break down over time at a known rate, offering us the ability to read a built-in clock. For example, radioactive decay from the parent-daughter pairs Re-Os, Rb-Sr, and Sm-Nd are all used in diamond dating, from inclusions such as iron-nickel sulfide, clinopyroxene, and garnet. Given that only some inclusions are appropriate for this kind of analysis and the process is destructive, it is often challenging to date the diamonds from any given deposit. A comprehensive introduction to diamond dating can be found in Smit and Shirey (2019).
LARGE-SCALE PROCESSES OF DIAMOND FORMATION
A key observation from decades of diamond studies is that diamonds can form in multiple distinct ways involving different host rocks, carbon sources, and diamond-forming reactions (Shirey et al., 2013). Interestingly, many of these diamond-forming processes appear to be linked to large-scale movements of solid rocks and fluid phases, such as the formation of continents and the release of melts and volatiles from tectonic plates as they sink and subduct into the mantle (e.g., Shirey and Richardson, 2011; Walter et al., 2011; Weiss et al., 2015; Shirey et al., 2024). Cross sections of the earth illustrating this activity often depict regions stretching hundreds or thousands of kilometers wide (figure 3).
In most cases, diamond growth in the mantle is said to be metasomatic, meaning it occurs as mobile carbon-bearing fluids invade preexisting solid host rocks and undergo changes that cause carbon to crystallize into a solid form. One of the most studied fluid types has been COH fluids (mixtures of carbon, oxygen, and hydrogen), which in simple terms can be thought of as a watery fluid mixture dominated by the molecules H2O (water), CH4 (methane), and CO2 (carbon dioxide). Diamond formation in the lithosphere could occur from such a fluid as the temperature and pressure decrease when it percolates upward through mantle rocks by the following chemical reaction (Luth and Stachel, 2014):
CO2 + CH4 → 2H2O + 2C (solid diamond)
Changing conditions force the fluid-borne carbon in carbon dioxide and methane to recombine into solid native carbon as diamond. Other diamond-forming fluids thought to be relevant to diamond growth range in composition from hydrous, silicic, carbonatitic, and saline to potentially even metal-sulfide melts (Luth et al., 2022). Perhaps the most curious diamond-forming medium is the Fe-Ni-C-S metallic melt found as inclusions in some CLIPPIR diamonds, a sublithospheric variety that includes most top gem-quality type IIa diamonds (Smith et al., 2016). CLIPPIR diamonds are anomalous in being the only gem-quality diamonds that contain large (>10 μm) inclusions of the growth medium. Coming back to the sense of spatial scale, CLIPPIR diamonds are an impressive example of large-scale movements. These crystals grow from ingredients delivered by descending oceanic plates that have been altered by seawater at the ocean floor and then subducted down to the mantle transition zone and the top of the lower mantle at 660 km (Smith et al., 2016, 2021). After growth, these diamonds are carried upward, potentially within low-density packages of rock derived from the subducted slab that rise under their own buoyancy. They may reside at the base of the continental lithosphere for an extended period (Timmerman et al., 2023) before later being drawn up to the earth’s surface in a kimberlite.
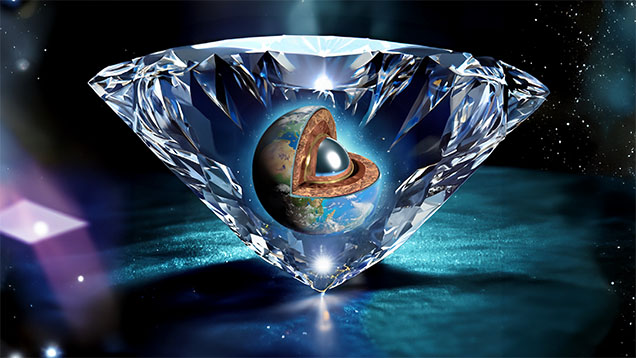
It is no coincidence that diamond is linked to large-scale processes. After all, diamond is made of carbon, which plays an important role in lowering the melting point of mantle rocks, facilitating partial melting and the generation of magmas. Carbon’s concentration in the mantle hovers around a few hundred parts per million but varies considerably with geological processes, such as volcanic activity. Carbon can also be introduced to the mantle by subducting slabs, both in sediments and altered oceanic crust. The balance of carbon entering the mantle through subduction and leaving the convecting mantle as magmas, volatiles, or metasomatic fluids forms the deep carbon cycle, which is critical in regulating atmospheric carbon dioxide over million-year timescales. The behavior of carbon influences geochemical cycles, mantle dynamics, and tectonic processes, not to mention the earth’s ability to sustain life. Considering that ours is the only known planet with plate tectonics and an active deep carbon cycle, the formation of diamonds in the mantle may be somewhat unique to Earth. And peering into diamonds gives insights into the inner workings of our planet in a way that no other mineral can rival (figure 4).
MAGMATIC JOURNEY TO THE SURFACE
While the multifarious crystallization of diamonds in the mantle is remarkable, yet another independent mechanism is responsible for bringing them up to the earth’s surface. Early diamond mining in India, Indonesia, and Brazil was all alluvial, meaning that loose diamonds were extracted from river sediments or other sedimentary deposits. These diamonds were plucked clean from their primary sources by weathering and transported and mixed with assorted grains, obscuring their mantle origins. In 1867, alluvial diamonds were discovered in South Africa along the banks of the Orange River, which set off a diamond rush that led to the discovery of weathered primary deposits in 1871 (Field et al., 2008). The new diamond-bearing igneous rocks became known as kimberlites, after the nearby town of Kimberley.
Kimberlites, and to a lesser extent lamproites and other related rocks, represent the most deeply derived magmas to erupt at the earth’s surface. Although many details remain unresolved, they are thought to be produced by low-degree partial melting in the asthenosphere, which produces a carbonate-rich magma with an evolving composition en route to the surface (Giuliani et al., 2023). A high initial content of volatiles in the melt, chiefly water and carbon dioxide, serves as fuel to help crack overlying rocks and propel the magma to the earth’s surface. The release of gases increases dramatically as the ascending magma approaches the surface. Over the roughly 200–300 km journey, the melt rips up pieces of rock and crystal grains, including diamonds occasionally, and may reach the surface rapidly, in a matter of just hours to days. With the element of chance involved, and considering the relatively high density of diamonds and mantle rocks as well as the fact that the magma threatens to oxidize or graphitize diamonds along the way due to the change in pressure and temperature, it is impressive that any diamonds make it all the way to the surface.
Diamonds are transported great distances vertically from the original settings in which they formed. Taking this into account helps explain the challenge of determining a diamond’s geographic origin. Multiple layers of evidence from diamonds and their inclusions show considerable overlap in the conditions of diamond formation from one locality to the next on a worldwide basis. Unfortunately, this reality means there may not be any geographically distinct features that would allow scientists to analyze a diamond and ascertain its country or mine of origin (Smith et al., 2022).
RARITY
The popularity and availability of gem diamonds combined with some persistent myths have left some people wondering how rare natural diamonds really are. In terms of its natural abundance on earth, diamond is a relatively rare mineral. Prospectors and geologists who make a career searching for diamond deposits tend to come up dry. Considerable ongoing expenditures by mining and exploration companies to locate new deposits would be foolish if not for their rarity. Only through modern mining practices responding to global demand have diamonds become as available as they are today.
In total there are about 7,000 known kimberlites and related mantle-sourced magmatic rocks worldwide, but only about 1,000 are of potential economic interest for diamonds (Kjarsgaard et al., 2022). Of these, even fewer have the right combination of size, quality, and abundance of diamonds as well as favorable logistical factors that would make mining feasible (such as the deposit’s depth, shape, and proximity to infrastructure). The question is always whether the sale of mined diamonds will actually outweigh the costs of exploration, mining, and reclamation and remediation when operations cease. Altogether there are fewer than 100 productive diamond mines past or present globally (Kjarsgaard et al., 2022). At most of these localities, the abundance of diamonds is still so low that they are almost never seen in the kimberlite—they only become visible at the final stages of ore processing. A decent ore grade is in the neighborhood of one carat per tonne, meaning a bathtub full of precious diamond-bearing kimberlite would contain on average one carat total weight of rough diamonds. More often than not, a tonne of ore contains multiple small diamonds, while seldom does it contain a gem-quality diamond exceeding one carat.
It is helpful to put diamonds in perspective by comparing them to another rare and precious material mined on an industrial scale: gold. By weight, about 125 times more gold is mined annually. Furthermore, only about one-third of the 110–130 million carats of diamond mined each year is actually of gem quality, with larger and higher-clarity diamonds being the scarcest. Global diamond production fluctuates year to year as mining output varies. The end of mining at Argyle in Australia in 2020 impacted the market, as will the expected end of production at Diavik in 2026. For now, the only new mine of similarly large scale is the Luele mine in Angola, which began production in 2023. Although diamonds may be actively forming deep in the mantle, it is important to recognize that the only diamonds available to humanity are the ones already at the surface. They are inherently finite in supply, and only a limited number of deposits are feasible to mine.
MINING AND ITS IMPACTS
Our world is underpinned by mining, an enormous industry largely hidden from view. Diamond mining has on several occasions entered the spotlight due to human rights concerns. In 2003, the Kimberley Process was established as an international certification scheme to prevent “conflict diamonds” from entering the mainstream rough diamond market. This has been a positive step forward, but it is not without challenges. Approximately 15% of global diamond production is from small-scale artisanal mining, and the people involved in these operations are most at risk of exploitation, violence, unsafe working conditions, and other problems. There are several corporate or industry initiatives focused on supporting and empowering artisanal diamond miners and communities, including Diamonds Do Good, the Diamond Development Initiative, Root Diamonds, and GemFair.
Less attention has been paid to some of the positive social impacts of diamond mining. Botswana is a great example. In 1967, only a year after Botswana gained independence from British rule, a large diamond deposit at Orapa became a beacon of hope for one of the world’s poorest countries at the time. Several additional deposits were soon found, and Botswana formed a partnership with De Beers. Today, Botswana is the top diamond producer by value, with the revenues providing significant economic benefits such as job creation and funding for critical infrastructure and social programs. The prudent management of diamond revenues has facilitated economic diversification, contributed to political stability, and positioned Botswana as a model for resource-rich developing countries.
In addition to social impact, diamond mining has an impact on the environment. Marketing efforts portray laboratory-grown diamonds as a “green” alternative to mined diamonds, but this is not a straightforward comparison. Both have environmental impacts, but their impacts are much different. Diamond mining is not as detrimental as many other forms of mining. Diamonds are extracted from rocks without the need for refining and smelting, as is the case for extracting copper or nickel from sulfide minerals, for instance. Diamond ore from a mine is crushed and diamonds are extracted mechanically, so the primary waste material is crushed rock. The recovery process uses water but few additional chemicals, if any. Curiously, the mineralogy of kimberlites is such that the weathering of this rock after crushing actually consumes carbon dioxide, with the potential to partially or even completely offset the carbon dioxide released by the mine (Mervine et al., 2018).
Artisanal diamond mining involves recovery from rivers or nearby sediments. The area affected by mining activity may be larger, but the depth that miners can reach with hand tools is limited to a few meters and the landscape is often reclaimed by vegetation when mining ceases. Larger mines targeting large alluvial deposits or primary deposits leave a larger footprint, typically some 20 to 30 square miles, often situated in remote areas. When a diamond mine closes, current best practices aim to restore the land to as natural a state as possible. The Argyle mine, one of the world’s largest diamond mines, closed in 2020 after 37 years of operation. The owner, Rio Tinto, has a responsibility to reclaim and remediate the mine site, which involves dismantling the infrastructure, reshaping the land, and restoring vegetation. They are not permitted to simply abandon the site.
The Victor mine in Canada is another example of a recently closed diamond mine undergoing remediation. Victor was a relatively small but high-grade deposit. Mine construction began in 2006, and by 2019 the mining and processing was complete. With the infrastructure now demolished, rehabilitation of the site is well underway. The open pit has been transformed into a lake, and 1.4 million native trees and shrubs have been planted. For their remediation work at Victor, De Beers was recognized with the 2022 Tom Peters Memorial Mine Reclamation Award, presented by the Canadian Land Reclamation Association. Obviously it is impossible to truly “undo” damage to the environment, but the legally mandated steps taken by modern mining companies substantially reduce the long-term impact of diamond mining.