On the Origin of Digit Patterns in Gem Opal
ABSTRACT
Some natural opals, mostly from Ethiopia, show a macroscopic finger-like structure called a digit pattern. This pattern consists of vertical columns that are more or less parallel, separated by a homogeneous matrix of different color, transparency, or play-of-color. This study proposes that digits develop through: (1) the deposition of a homogeneous opal layer and subsequent polygonization in the form of vertical columns; (2) preferential alteration of this layer at the vertical grain and sub-grain boundaries, creating the digit shape; (3) precipitation of a new silica gel in the space between the digits; and (4) the drying and solidification of the opal. Although polygonization in the form of vertical columns is a growth process typical of synthetic opal, the post-growth alteration of these columns into digits and the deposition of matrix are observed only in natural opal.
Opal is a poorly crystallized or amorphous hydrated silica formed through the solidification of a silica gel (Jones and Segnit, 1971). The most valuable variety is “precious opal,” which displays play-of-color: patches of pure spectral colors from violet to red flashing over the stone as it is tilted. Precious opals are mined in many parts of the world, most notably in Australia, Brazil, Mexico, and Ethiopia. Other sources include the United States, Honduras, and Java. Ethiopia has been a major producer since the 2008 discovery of abundant opal at Wegel Tena, in the northeastern Wollo Province (Rondeau et al., 2009, 2010; Mazzero et al., 2009, 2010).Play-of-color arises from the diffraction of visible light on monosized, well-ordered silica spheres in opal-A (Sanders 1964; Darragh and Sanders, 1965), and lepispheres in opal-CT (Flörke et al., 1976) of appropriate diameter. Most often, play-of-color involves several juxtaposed patches of various diffraction colors. In rare cases, a network of silica spheres is distributed over the whole stone, so that the color patches move in unison. Such samples are considered natural “photonic” crystals. Whereas a crystal sensu stricto diffracts X-rays, a photonic crystal diffracts wavelengths in the visible range of the spectrum, giving rise to visible play-of-color. The diffraction colors in precious opal can be arranged in a variety of patterns, including intense specks (pinfire), flames, bands, and juxtaposed polygons (harlequin opal). Straight black lines or bands often cross the patches, and these are due to polysynthetic or mechanical twin planes of the photonic crystal, merging at the surface of the gem (Kinder, 1969; Gauthier, 1985). Unlike precious opal, common opals do not display play-of-color, usually because the silica spheres lack regular packing (Gaillou et al., 2008).
Although some rare opals are colorless, most specimens present a bodycolor: white, black, gray, brown, yellow to orange (as in fire opal), red, pink, blue, green, or violet. These colors are typically due to minute mineral inclusions colored by transition metal ions that absorb part of the visible spectrum of light. These include iron for yellow to orange to brown (fire opal), copper for a saturated blue (“Peruvian opal”), and nickel for green (chrysopal; Fritsch et al., 1999). Other causes of color in the inclusions are color centers (purple fluorite inclusions) and organic compounds (quinones in pink opals; Mathey and Luckins, 1998; Fritsch et al., 2004).

Digits are so frequently observed in Ethiopian opals, either from Wegel Tena in Wollo Province (Rondeau et al., 2010) or from Mezezo in Shewa Province (Johnson et al., 1996; Mazzero, 2003) that they have become the industry’s unofficial identifier for Ethiopian opal. Examples have occasionally been reported from other deposits, such as Virgin Valley, Nevada (Gübelin and Koivula, 2005; Gaber, 2007). Digits have been reported in only one Australian sample (figure 2). This paper aims to provide further documentation on digit patterns by proposing a model for their formation.
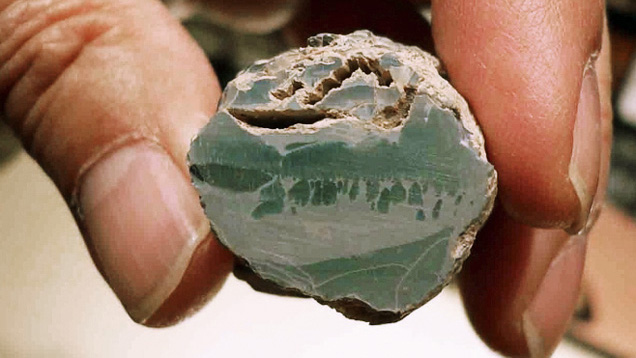
MATERIALS AND METHODS
All Ethiopian samples were rough or cabochon opals collected in the gem market at Addis Ababa, Ethiopia, between 2008 and 2011. Their digit patterns were observable with the unaided eye at the millimeter or centimeter scale (again, see figure 1). From thousands of samples examined, more than 10% of them showed digit patterns. We documented those that showed spectacular or interesting features before they were sold. The specimen from Honduras was photographed during the 2011 Sainte-Marie-aux-Mines gem and mineral show (see figure 14). The specimen from Australia (figure 2) was photographed at the Coober Pedy mine.We photographed the specimens using a camera equipped with either a macro objective lens or a binocular microscope with up to 50× magnification.
RESULTS: OPTICAL PROPERTIES OF DIGIT PATTERNS
As defined earlier, digits are characterized by their shape and revealed by the optical contrast between the columns and the matrix. This section provides observations and interpretation useful for establishing a formation model.Variation in the Appearance of the Digits. In samples that contain abundant matrix and few play-of-color patches, the digits are clearly rounded at the ends (again, see figure 1). In samples that contain less matrix and abundant patches, the digit ends are less rounded and more polygonal (figure 3). Some samples display juxtaposed, polygonal columns with no matrix at all in between. In this last case, the digit pattern is no longer visible, and such stones show, in a transversal section, juxtaposed polygonal columns of play-of-color opal (as in the lower right part of figure 3). This is somewhat similar to the harlequin opal.

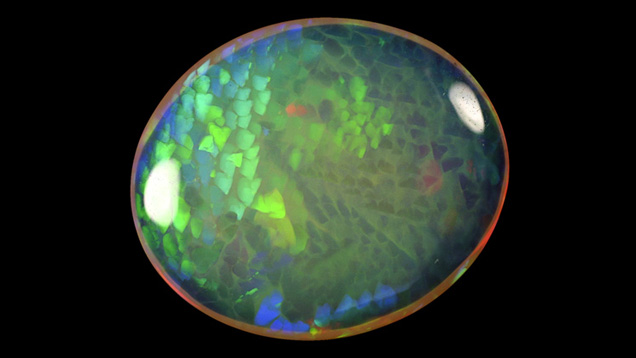
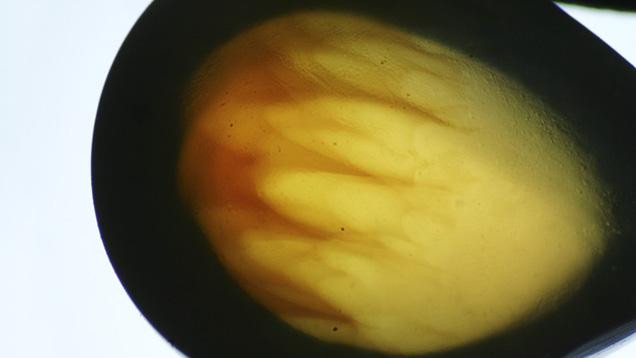
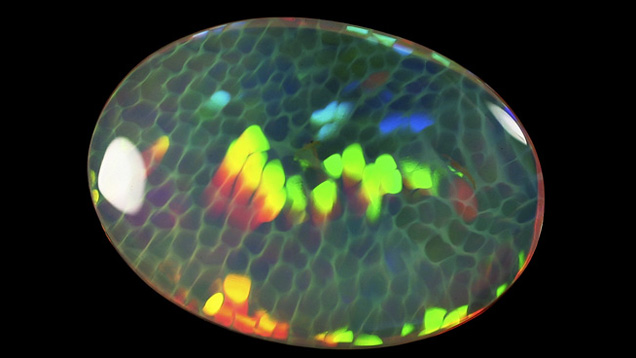

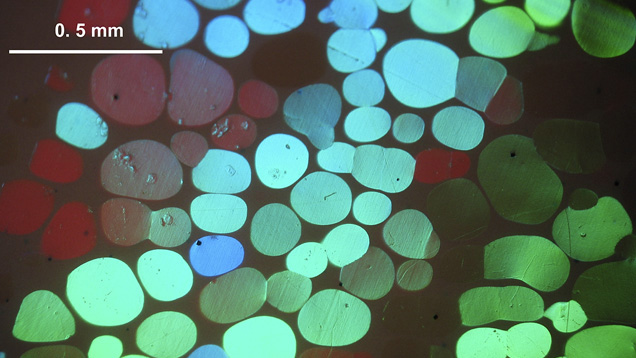




DISCUSSION
A Model for Digit Formation. Based on the previous observations, we propose a step-by-step model for digit formation, illustrated in figure 13. This model deals specifically with the development of digits in play-of-color opal.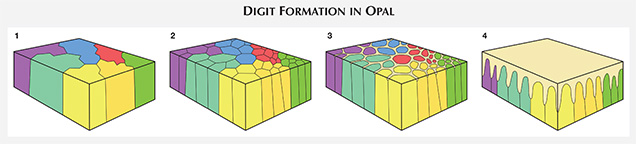
- Step 1: Formation of Play-of-Color Opal in Silica Gel. Hydrated spheres of silica combine to form either a single large, homogeneous photonic crystal or several juxtaposed ones, forming prismatic, vertical columns.
- Step 2: Polygonization of the Crystals. Photonic crystals split into juxtaposed columns, polygonal in cross-section (which we refer to as sub-grains). Each column generally has five to six faces per prism, but may have four or seven in rare cases.
- Step 3: Remobilization of the Photonic Crystal by a New Fluid with Opal Etching Properties. Alteration of the photonic crystal by remobilization of the silica spheres occurs preferentially at the grain and sub-grain boundaries, which offer more available surface. Individual columns (either grains or sub-grains) then acquire their rounded shape, forming true digits.
- Step 4: Deposition of Common Opal. Changes in local chemical conditions lead to deposition in the remaining spaces between digits. When step 3 is more pronounced, so is step 4.
- Step 5: Additional Deposition. Additional horizontal layers of opal of any variety may also be deposited.
- Step 6: Solidification. Through dehydration and cementation, this silica gel forms a solid opal.
How Does Polygonization Occur? Polygonization (step 2) also occurs in synthetic opal. It happens after the silica spheres are deposited and while the silica gel is drying (Nassau, 1980). Polygonization may be due to the bonding forces between the gel and the walls of the cavity that contains the gel. Alternatively, it may occur because of the expulsion of the liquid from the silica gel (dehydration), producing a contraction effect, a phenomenon known as syneresis. Syneresis should create some space between the columns, but this was not observed in our samples. In either case, columns are vertical because there is a free horizontal surface at the top of the gel. Polygonal columns in opal evoke the basaltic prism that forms due to shrinkage during magma cooling.
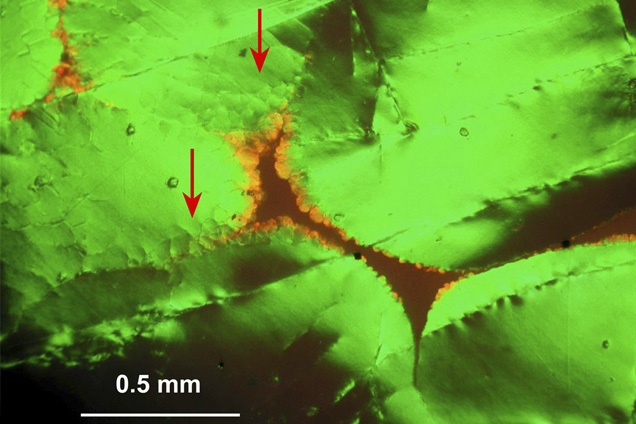
How Does Remobilization (Step 3) Occur? Similar rounded shapes are found in many crystals in nature, such as beryl or diamond. Those are generated by dissolution processes (Sunagawa, 2005). This happens when the growth medium turns unfavorable due to changes in temperature, pressure, oxygen fugacity, redox conditions, chemical potential, or other variables. In the case of opal, remobilization of the silica spheres is enough to cause erosion of the photonic crystal, rather than true dissolution. This may occur when an influx of water with a lower silica content leads to the remobilization of the previously deposited silica. In samples that are well separated by abundant matrix, and have thus undergone more significant alteration, the digits show a more rounded shape.
Variations on the Model. All these steps can occur in a single sample, in particular when alteration (step 3) only affects the upper part of the columns. More often, some steps are not seen because the sample was cut or broken during mining. For example, the stone in figure 3 shows only steps 2 (polygons of play-of-color opal) through 4 (rounded patches separated by some matrix). Likewise, figure 11 shows the result of steps 4 and 5 only. Patterns formed by only steps 1 and 2 are common in opals from many deposits, including Australia (Pecover, 2010), and those in thin fractures in basalts from Honduras (figure 15). Such samples show a “honeycomb” structure (Maddison, 1992) somewhat similar to that observed in synthetic opal, in which case digits did not develop because no remobilization stage occurred.
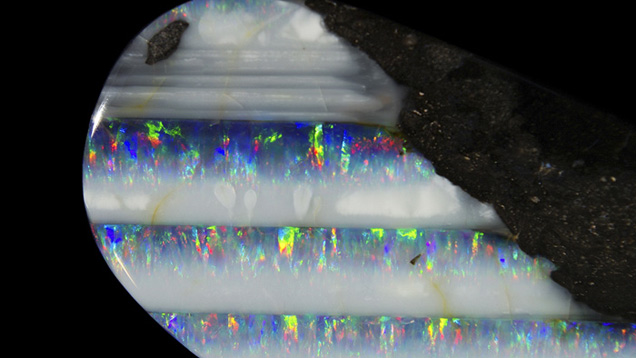
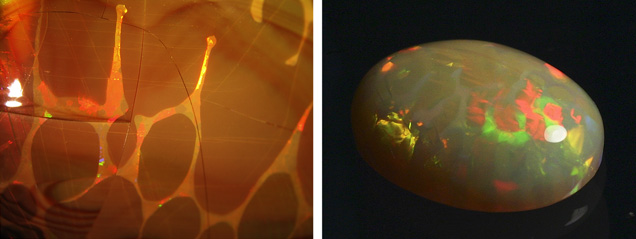
Confusion with Synthetics. Ethiopian opals showing the “lizard-skin” effect are not uncommon, as in the bottom right area of the stone in figure 3. This may cause confusion with synthetic opals (e.g., Choudhary, 2008). The presence of a matrix between the columns may therefore be the first step in identifying a natural opal. When no matrix is present, other indicators are inclusions typical of Ethiopian opals, such as opaque black octahedra, pyrite cubes, or rootlet fossils (Gauthier et al., 2013).
CONCLUSION
This study has documented the macroscopic characteristics of digits, distinct aesthetic features observed in many natural opals from Ethiopia but rarely seen in specimens from other regions. We propose that these columns form vertically with their rounded ends pointing upward, and that larger patches of color formed before the matrix. The first step in our model is the deposition of a homogeneous layer of opal with juxtaposed vertical columns. Next, polygonization of the columns occurs. Then, an influx of fluid (probably less rich in silica) remobilizes this layer. The remobilization is most efficient at grain boundaries, separating the columns and rounding their edges. Finally, opal precipitates again between the partially dissolved columns, cementing them into a matrix of either common or play-of-color opal.Among the open questions remaining, we emphasize that the process by which remobilization occurs remains unclear: Is this a true dissolution process, in which silica spheres dissolve into water, or do the spheres remain intact but dispersed into the gel? Also, the combination of geological conditions necessary for digits to form have been encountered in Ethiopia but are uncommon in other localities: What makes this combination so rare?